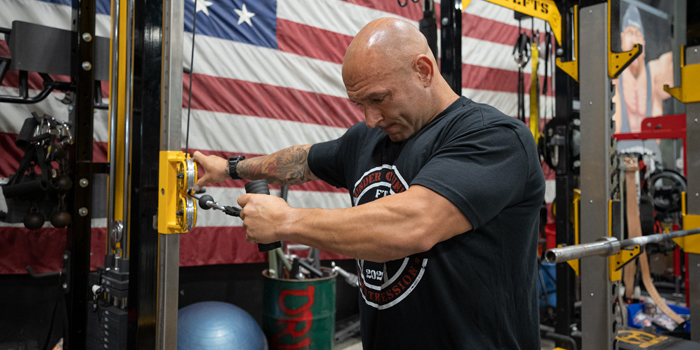
Skeletal Muscle Contractions
A skeletal muscle contraction begins when the nervous system generates a signal called an action potential. This action potential travels along the nerve cell called a motor neuron. Once it arrives at the nerve terminal, it depolarizes that terminal. This activates voltage-gated calcium channels. The increase of calcium causes a release of acetylcholine which is stored in the vesicles and is released into the synaptic cleft through exocytosis.
Acetylcholine binds to the post synaptic receptor nicotinic-cholinergic receptor (NAChR). Activation of the NAChR allows diffusion of sodium into the cell and potassium out of the cell. At this endplate you have acetylcholine esterase (AChE). AChE hydrolyses the Ach to choline and acetate. This transmission is about 1ms. The membrane surrounding the endplate is electrically excitable because it contains voltage gated sodium and potassium channels.
This current that is produced spreads to the surrounding muscle membrane. It depolarizes the muscle membrane to threshold and activates the voltage-gated sodium channels and produces an action potential. The action potential travelling down the sarcolemma from the end plate enter the T-tubules and activate voltage gated calcium channels. This activates calcium release channels causing a release of calcium from lateral sacs of the SR.
Calcium that is released then binds to troponin c removing the blocking action of tropomyosin. Myosin then binds to actin and cycles, calcium ATPase pumps calcium from the cytosol into the SR. This decreases the cytosolic calcium concentrations. Calcium dissociates from troponin and cross bridge cycling ceases. The sarcomere extends back to its resting length.
Sliding Filament Theory
The sliding filament theory is a model that explains contraction force generation that produces shortening of a skeletal muscle fiber. It states that the ability of a muscle fiber to generate force and movement depends on the interaction of the contractile protein’s actin and myosin. That these filaments overlap in each sarcomere and propel by the movements of the cross bridges.
Myosin is a protein that converts ATP into mechanical energy, which creates thrust and movement. Actin has myosin binding site which are revealed when troponin is removed via calcium binding to it and this facilitates a bridge formation between actin and myosin. This theory seems to hold to true for contractions and isometrics but during the eccentric portion of the lift it may not be so relevant.
It does not predict eccentric muscle contractions well, produces instability of myosin alignment and force production on the descending limb of the force-length relationship, and can’t account for the vastly decreased ATP requirements of actively stretched muscles (Herzog et al 2012).
Three-Filament Model
The three-filament model includes titin into the mix due to its prominent role in the eccentric portion of the lift. Titin is an active participant in muscle force regulation by changing its stiffness in an activation/force dependent manner and by binding to actin and by doing so it adjusts its free spring length. Myofibrils passive force production is governed almost exclusively by titin which spans the half sarcomere from z disk to m band. It functions as a molecular spring that elongates when sarcomeres are stretched, developing passive forces (Tilp et al. 2015).
Muscle Protein Synthesis
Signal transduction is a hypothesis for predicting muscle growth and it says specific sensor proteins detect exercise related signals, which are then computed by transduction pathways or networks. These regulate downstream events including gene transcription, translation or protein synthesis and protein breakdown.
For a gene to be expressed as a protein the DNA needs to be transcribed into RNA. RNA becomes mRNA through a process called splicesomes. mRNA then gets translated into a protein. The mechanisms of protein synthesis include translation of mRNA combined with the post transitional processing and transport of polypeptide products.
Protein synthesis can be increased in the short term by increasing the translational efficiency and in the long-term by increasing the translational capacity. There are two general mechanisms for translation initiation and they are cap dependent and internal ribosome entry site (IRES).
How it Works
Cap dependent translation is regulated by two processes which are eIF2 and eIF4F. eIF4F interacts directly with the mRNA 5’ cap structure and recruits 40S ribosomal subunits to it. eIF2 binds to GTP Met-tRNA and forms the ternary complex which then binds to 40S and leads to the 43s-preinitiation complex.
This complex scans the mRNA for its initiator codon and binds to it. GTP is then hydrolyzed to GDP by eIF2, leading to the release of eIF2 GDP and other components, and binding of 60s. This leads to growth, survival, motility, and division. The more ribosomes we can produce the more protein synthesis can occur and this is done by resistance training.
mTOR is a key mediator of muscle hypertrophy following resistance training. mTOR regulates and promotes muscle hypertrophy via S6K through the regulation of cap dependent translation and an increase of the translational machinery. S6K phosphorylates a number of initiation factors helping to form the initiation complex which enhances translation efficiency and cell growth.
References:
Schappacher-Tilp, G., Leonard, T., Desch, G., & Herzog, W. (2015). A novel three-filament model of force generation in eccentric contraction of skeletal muscles. PloS one, 10(3), e0117634. https://doi.org/10.1371/journal.pone.0117634
Herzog W, Leonard T, Joumaa V, DuVall M, Panchangam A. The three filament model of skeletal muscle stability and force production. Mol Cell Biomech. 2012 Sep;9(3):175-91. PMID: 23285733.
BONUS: My video on breathing right to lift more.