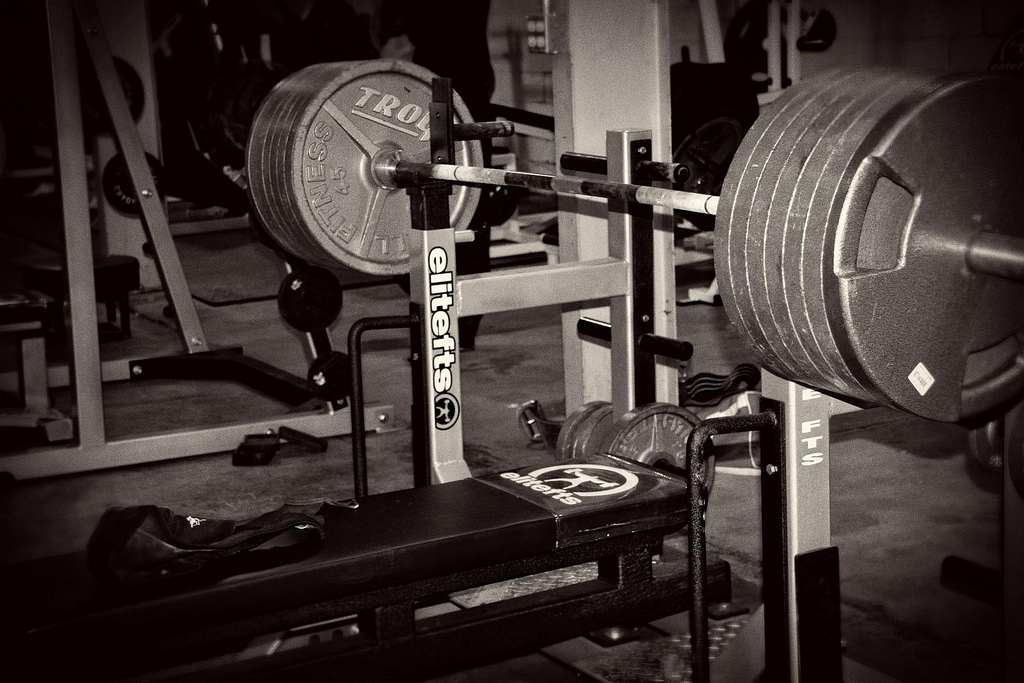
Overview
The Westside Method is perhaps the best example of strength program that requires its user to have some knowledge of physics, biology, anatomy, and specialized sport science. The following series is intended to give new lifters some general information on major aspects of weightlifting science.
Part I: Strength and the Body
Part II: Methods of Strength Development
Part III: Periodization: History and Early Models
Part IV: Advanced Periodization Models
Strength and the Body
From a practical perspective, strength is the body’s ability to generate force. It is created through a complex interaction between the nervous system, skeletal muscles, bones, and energy systems. To understand how to maximize the neural and muscular components of strength, a little background on the body can be useful before moving on to methods and arrangement. Our knowledge of these two systems is incomplete, though the following aspects are generally agreed upon.
Nerve and Muscle
Nerve cells are called neurons. Like most cells, their hub is a round structure that contains a nucleus and other structures necessary for communication, growth, repair, and division. Where neurons differ from the average cell is that they possess long, branching tubes that are filled with cellular fluid; when viewed through a microscope, it looks like the nucleus has roots. The fluid, cytoplasm, conducts electricity, which is important since every movement message in your body is sent via electro-chemical pulse. An inert membrane forms the structure of the tube and prevents the current from leaking. Because much of the human body is made of cytoplasm, the coating is essential to make sure that signals traveling back and forth along the nerves get to their intended location.[i]
It’s no surprise that nerves are often compared to electrical wiring. Both conduct electrical currents and have conductive cores surrounded by a sheath; this correlation actually leads neurological science to look like a cross between biology and engineering. A more specialized definition might be to compare a nerve to an underwater internet cable: much like a nerve, such a cable carries information through a highly conductive environment to distant end destinations that actively receive and propagate these signals.
Muscle consists of sequential rows of protein-heavy elements that contract when signaled, and it is the regulated contraction and relaxation of these cells that leads to movement. Muscle cells are unusual, which means a lot of what we know about typical cells gets thrown out the window. One key way they’re odd is that they’re multinucleated. This means that unlike our neurons from earlier, muscle cells have more than one nucleus, and these multiple nuclei seem to be both connected to sarcoplasm[ii] (a nutrient-rich cousin of cytoplasm) and essential to hypertrophy, as they divide before a muscle actually grows.[iii] Another key difference is that muscle tissue also has partial cells called satellite cells, which are single-nucleus cells that repair muscle damage and seem to be excited by a lot of the same hormonal activity associated with training and hypertrophy. These cells fuse into complete muscles cells, which is why the full cell is multinucleated.
The major structures of the muscle look roughly similar—long and ropey, with all of their elements running in the same direction—so it’s a bit like your muscles are Russian nesting dolls of protein threats. Down at the micro-level of muscle are contractile elements called sarcomeres, which are stacked into strands myofibrils, which are grouped together into the actual muscle cell or fiber. The fibers are themselves grouped together into fascicles, and these are collected into a full muscle like a pec or hamstring.[iv] Sarcoplasm surrounds the fibers and helps provide the muscle with nutrients for energy and repairs.
Neurons that reach out into these muscle fibers are called motor neurons; a motor neuron and all of the muscle fibers it touches is called a motor unit. This is important to know because when a motor neuron sends a signal to its muscle fibers, it causes each fiber to contract. Very roughly, there are two kinds of motor units: fast-twitch and slow-twitch. Fast-twitch units are large, used for forceful movements, have low endurance, and are only used when needed; slow-twitch units are smaller, are used for low-strength movements, don’t fatigue quickly, and are used in all movements. There is actually a continuum between the two types, and the units can change characteristics depending on the activity they’re exposed to.[v] This means that lifting heavy weights will cause slow-twitch units to behave more like fast units, while practicing for a marathon will cause faster units to act more like slow units.[vi]
This is just a snapshot of muscle cells—I imagine that before it’s all said and done, there’ll probably be a dozen or more distinct pathways and processes that all work together in a knotted mess to make muscles larger. If you’re interested in learning more about the micro-level stuff, look up IGF-1, myosin heavy chain expression, and the mTOR pathway. You should be busy for a while.
Neural Strength
When it comes to movement, the brain doesn’t have a “lift this slowly signal” or a “lift this explosively signal” where a brain message of x millivolts lets you gently pick up your child, and a stronger signal of y millivolts causes you to fling him through the ceiling. Motor units actually respond to the frequency of the nerve impulses they receive. This means that most of our movements aren’t completely smooth actions, but the result of millions of microscopic mini-contractions within our muscle fibers. The less time the fibers take between a mini-contraction the stronger the entire muscle contracts. When the muscle is actively shortening, this is called the concentric portion of a movement; when the muscle is static, this is an isometric contraction; and when the muscle is lengthening, the movement is called the eccentric portion.
Motor units are also recruited from the smallest available unit to the largest as the frequency increases. Imagine a man taking a leisurely walk. He could keep up the walk for hours using only slow-twitch motor units, which burn stored body fat for fuel. If he wants to move to a quick jog, the brain sends a faster series of signals to the muscle which causes slightly faster slow-twitch units to be brought in. If he picks up the pace, the signal rate climbs and even faster units are added; these use glycogen for fuel. Finally, if the jogger breaks into running so that after a few seconds his heart feels ready to explode, the signal rate increases to the point that all the motor units are moving, with the freshest units using small ATP and creatine phosphate stores for fuel. If he starts a full-on sprint or explosively jumping, there aren’t any additional motor units to recruit; the brain compensates for this by increasing the signal frequency even more.
Our example above showed two ways the nervous system creates strength. Recruitment is the act of enlisting a greater number of motor units, and can occur up to about 80% of person’s maximum effort at a given activity. Rate coding is an increase of signal frequency, which can continue increasing even after all of a muscle’s motor units are active. A third method, synchronization, exists beyond these two. Normally, motor units are activated to produce a smooth movement, though high-level athletes have the ability to simultaneously contract all their fibers, causing a sudden burst of power.[vii],[viii] This synchronization appears to have been reduced in humans as a course of evolution; compared to closely-related mammals, humans have superb endurance but poor maximal strength, as well as additional structural deficits and inhibitory mechanisms that hamper our efforts at meeting our strength potential.
Before moving away from the nervous system, I want to talk a little about movement. The super-simple example of strength from the beginning could be made more specific to athletes by saying that strength is the body’s ability to produce force as part of creating and/or resisting movement. Imagine the squat: while the leg muscles act upon the femur, tibia, and pelvis to create movement (raising and lowering the bar), the abdominal and spine muscles are fighting to resist movement in the spine (unwanted flexion).
The learned movements are called motor skills. Strength is nothing without the guidance of movement, as the body can’t apply force without first being rehearsed in its movement. Lifters experience this in a very short-term manner when warming up; even though the involved muscles should be fatigued after the first warm-up reps, performance of a lift will actually improve as the nervous system re-grooves the pattern. The importance of motor skills is just as evident when you try a new exercise (or even a new load for a familiar exercise) and find that your reps go up with every set despite being fatigued from the earlier reps. When applied to moving weights, lifters refer to motor skills broadly as technique. Technique is often referenced as an individual act, but not so much as a component of strength or strength programming. I hope to address that as this series continues, because the Westside method is very smart in how it grooves technique.
Muscle Strength
All other things being equal, a bigger muscle is a stronger muscle. Just how the muscle gets bigger isn’t quite as clear, which goes back to our earlier look at muscle anatomy, as the way other cells work doesn’t carryover as much. Whatever triggers it, there are two main types of muscle growth: hypertrophy is the process of enlarging existing muscle fibers, while hyperplasia is the act of growing new muscle fibers. Hyperplasia seems to be the lesser of two forms.[ix] Several studies indicating statistically significant hyperplasia after weight training were muddled by chemical use.[x] You’ll also hear about old studies referencing fiber-number differences between bodybuilders and powerlifters, though as above, these are muddled by androgen use or are likely to represent inherent genetic differences between the different kinds of lifters. It seems hyperplasia could happen in an average Joe, but not to a significant extent, and its appearance is linked to standalone hormonal processes.
Hypertrophy has also been controversial. An older model of hypertrophy held that there were two methods of enlarging muscles: myofibrillar hypertrophy, which involved making actual muscle fibers larger; and sarcoplasmic hypertrophy, which involved increasing the volume of sarcoplasm around the muscle fibers. The theory went that lifting in the 1-5 RM range triggered useful hypertrophy that made the working parts of the muscle—the myofibrils—larger, which is why powerlifters appeared to be stronger than similarly-sized bodybuilders. Meanwhile, reps closer to the 10 RM range just swelled a muscle with cytoplasm, and had little effect on increasing the size of a muscle’s working parts, or of increasing its strength. The takeaway thought was that working in the 10 RM range (or with even less weight) had no positive effect on athletic performance.
Some older studies did back up the theory, though newer work downplays their conclusions. Of course, looking at the neural sources of strength, it’s easy to understand that a bodybuilder using 10-15 RMs (which are about 75% or less of a one-rep max) wouldn’t regularly be improving his rate coding or synchronization, or as forcefully engaging and converting his motor units towards the fastest end of the spectrum. On the other hand, strength athletes and recreational lifters alike have long used similar reps to get bigger and stronger, with biopsies on both populations showing fiber hypertrophy.
What seems more likely is that both bodybuilding and powerlifting rep ranges stimulate myofibrillar hypertrophy and neural strength gains. In addition to being connected to muscle fiber nuclei, sarcoplasmic hypertrophy may correlate to a combination of nutrient depletion, nutrient ingestion, and resistance training.[xi],[xii] It may be that sarcoplasmic hypertrophy is more connected with the metabolic demands of an activity than anything else, and may be largely absent in activities that don’t have great metabolic demands (such as a one-rep max bench press). The common factor of seemingly all effective strength programs is a general increase in the amount of total weight lifted over time. We’ll explore this topic better in later parts.
Before leaving muscles behind, an interesting physical aspect should be noted. If the eccentric portion of a lift is rapid enough, the stretched muscle unit can actually store energy like an elastic band.[xiii] This effect—known as the stretch-shortening cycle—is why pausing at the bottom of a lift is hard. “Bouncing” a squat out of the hole is an example of how to take advantage of the stretch-shortening cycle.
Looking Ahead
Just as complicated as the components of strength is how it is expressed and built-up. Part II of this series will examine different kinds of strength and the basic methods for improving it.
[i] Keynes, R.J. and Aidely, D.J. (2001). Nerve and Muscle: Third Edition. New York: Cambridge UP.
[ii] Allen, DL et al. (1999). Myonuclear domains in muscle adaptation and disease. Muscle Nerve, 22(10), 1350-60.
[iii] Bruusgaard JC, et al. (2010). Myonuclei acquired by overload exercise precede hypertrophy and are not lost on detraining. Proceedings of the National Academy of the Sciences of the United States of America, 107(34), 15111-6.
[iv] Ibid
[v] Zatsiorsky, Vladimir M. (1995). Science and Practice of Strength Training. Champaign, IL: Human Kinetics.
[vi] Vila-Cha, C. et al. (2010). Motor unit behavior during submaximal contractions following six weeks of either endurance or strength training. Journal of Applied Physiology, November,109(5), 1455-66.
[vii] Zatsiorsky
[viii] Schmied A., and Descarreaux M. (2010). Influences of contraction strength on single motor unit synchronous activity. Clinical Neurophysiology, 121 (10), 1624-32.
[ix] McCall, G.E., et al. (1996) Muscle fiber hypertrophy, hyperplasia, and capillary density in college men after resistance training. Journal of Applied Physiology, 81(5), 2004-12.
[x] McDonald, L. (2010). Categories of weight training: part II. Bodyrecomposition. Retrieved from http://www.bodyrecomposition.com/training/categories-of-weight-training-part-2.html.
[xi] Cuthbertson, D.J., et al. (2006). Anabolic signaling and protein synthesis in human skeletal muscle after dynamic shortening or lengthening exercise. American Journal of Physiology, Endocrinology, and Metabolism, 290(4), E731-8.
[xii] Moore, D.R., et al. (2009). Differential stimulation of myofibrillar and sarcoplasmic protein synthesis with protein ingestion at rest and after resistance exercise. Journal of Physiology, 587(pt. 4), 897-904.
[xiii] I use “muscular unit” here as a non-scientific acknowledgement that there is debate over whether energy storage occurs in the muscle belly or the tendon.
1 Comment