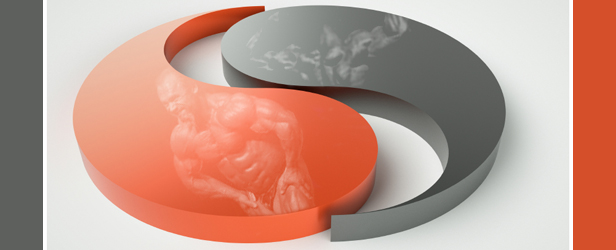
“If our brains were simple enough for us to understand them, we’d be so simple that we couldn’t" (1).
So where does this “muscle memory” reside? Is it furrowed deep in the nervous system (hidden away in our brains) or does the skeletal muscle itself have a way of remembering its past duties? It appears that the answer to that question is yes.
Tricky brains
Maximal muscle electrical activity (integrated EMG measurement), which indicates the nervous system’s capacity to activate muscle, does indeed decrease as strength is lost during detraining (2). However, this story of the nervous system’s role in detraining isn't so simple.
Perplexing clues about nervous system-related “muscle memory” come from unilateral training studies where a cross-training effect (the untrained limb actually gets stronger) persists during detraining (3). However, this effect varies among individuals (4). Naturally, untrained but “cross-educated” muscles aren't actually loaded in these cross-training studies, so the strength gains (and losses) suggest some sort of (centralized) learning effect. In one study, the greater the cross-training gains made, the greater the strength lost in the untrained limb during detraining. This relationship wasn't apparent, however, in the trained limb where muscular (de)adaptations also effect strength (4). In this study, muscle activation in the cross-trained leg wasn't correlated to strength changes during detraining (and some subjects even got stronger in the untrained leg). Thus, the authors suggest that something other than the central nervous system—something specific to the individual—may be involved with maintaining strength after training is discontinued. (Fascinating, eh?)
An interesting study from Finland bodes well for strength training in middle-aged and older newbies to the iron game and may help us better understand the nervous system’s role in detraining. When strength training carries over to “functional changes” like faster habitual walking speed and greater vertical leaping ability, these positive adaptations may persist for months, even when the training-specific strength and neural adaptations have faded (5). This suggests that the functional (neurological?) benefits of strength training in older individuals (6) may be maintained even when “detraining” has taken its toll (7) and that non-training activity may be involved in preserving some aspects of training adaptations.
Thrifty muscle
The extent of “disuse” following a period of training also plays a role in the deconditioning response of the skeletal muscle (8). In the lower body training study from Ohio University (see Part I), seven to eight months of “detraining” meant loss of size in the type IIB fibers, but there was also a reversal in fiber type percentages such that the IIA fibers not being used regularly converted back to IIB fibers (9). The type I and IIA fibers (those that didn't revert to IIB fibers) actually maintained their new-found size perhaps because the (now more fit) women were more active. Generally speaking, it's the type II fibers that show the greatest gains in size with resistance training (10–12) but also show the greatest atrophy with detraining (13). In other words, those fibers that aren't used with regularity are more susceptible to reversal of hypertrophy.
In cases of “extreme” detraining, such as immobilization (casting), muscular detraining can be even more rapid. Five to six weeks of immobilization are enough to more than reverse five to six months of training, resulting in fibers that are smaller than in the untrained state (14). (Still, more than half the strength gains may persist in this case (14), suggesting that the nervous system still “remembers” what it was taught.) Of course, biochemical adaptations to resistance training [e.g., increased glycogen content (15) and enzyme activities (13, 16)] will be general and gradually reversed over time as well.
Lots of “little brains” in really smart muscle
As noted in Part I of this article series, under normal circumstances when training for many months, hypertrophying muscle fibers add myonuclei as they grow. Even with immobilization, these nuclei appear to hang around for weeks apparently waiting in reserve if/when activity returns to normal (14). When the stimulus for muscle growth is extreme (e.g., in animal research), the incorporation of new nuclei actually precedes substantial muscle growth (17). However, if regular resistance training only lasts a few months, the process of incorporating these new nuclei (differentiation of the new satellite cells) may be cut short, leaving a proliferation of satellite cells that never end up within the growing muscle fibers (18, 19).
On the other hand, crafty scientists have shown that muscle cells can indeed grow substantially without increasing myonuclei number (20). However, when satellite cell proliferation (and differentiation) is blocked, so is the appearance of small fibers in rapidly growing muscle (20). An abundance of small fibers of this sort have been found in highly muscular bodybuilders, lending support to the notion that an increase in fiber number (hyperplasia) is important for developing tremendous overall muscle size (21, 22).
It appears that those hardy female subjects in the study at Ohio University who bombed their quads and hamstrings twice weekly with twelve sets to failure (9, 23) may have also been teaching their muscles a “lesson” in muscle memory by way of increasing myonuclei number.
Beyond genetics?
Beyond the increase in genetic material (more nuclei), alterations in how the genes themselves are expressed, without a genetic change (e.g., amputation) per se, appear to play a role in how muscle cells know (remember) how to adapt. The study of these mechanisms, called epigenetics, has blossomed recently and likely plays a role in the “muscle memory” phenomenon (24). Processes that involve how DNA is packaged with protein (e.g., chromatin remodeling or histone modification) or even adding methyl groups to the DNA itself play a role in satellite cell behavior (proliferation and differentiation) (25). These kinds of epigenetic processes are actually set in motion in skeletal muscle after just one exercise bout (26). Satellite cells from the same animal, although identical in DNA sequence, store an epigenetic “memory” of the muscle fiber type from which they were harvested (27). Indeed, it seems that the activity pattern (and simply the manner of innervation) during growth and maturation (24), in addition to the training stimuli a muscle encounters (28), pre-program these satellite cells to express contractile protein and behave metabolically like the muscle of their origin (27). In other words, satellite cells themselves store memory epigenetically in addition to leaving instructions in the form of (more) myonuclei within functioning muscle cells.
So gung ho from the get go (or not)?
So science has confirmed that “muscle memory” does exist, but I leave the ultimate decision to you, the reader, as to whether you (or your clients/athletes) should go “gung ho” when returning from a layoff. In summary, here are a few things to consider:
- A clever study recently found that muscle soreness and damage could be essentially eliminated by easing back into training for three weeks (“pre-training”) before a more focused eight-week training program. Both groups (the pre-trained and the “gung ho” group) improved strength and muscle size equally from start to finish (29).
- Muscle cellular disruption (damage) may be quite substantial during a period of retraining (9) but somewhat disassociated from measures of muscle pain (29, 30). (Be wary of overdoing it.) Massage is one of the few modalities to significantly reduce delayed onset muscle soreness in several studies (31–33) whereas non-steroidal anti-inflammatories (e.g., naproxen sodium) may help minimize muscle damage in some cases (34, 35) but not others (36, 37).
- A single soreness producing training session can produce a protective (“repeated bout”) effect against muscle soreness and damage the next time you hit the gym, an effect that can last for up to six months (38). Being resistance trained in the first place also reduces the damage incurred by a novel (eccentric contraction-based) bout (39). Thus, it’s quite possible that simply being (recently) weight trained confers some lasting protection (40) against debilitating soreness when you decide to hop back into the “iron saddle.”
- Several studies support the notion of retained muscle size and strength over many weeks or months of detraining as well as neural and muscular mechanisms of muscle memory that may speed your return to former iron heaving glory.
- The harder and longer one has trained, the more likely these memory mechanisms may be in place to slow the detraining process.
- Training-induced changes in day to day muscle activation or activity levels may also persist during detraining, slowing the loss of training benefits. A special advantage for older trainees may be changes in (daily) function outside the weight room that last for months when away from the gym.
- The greater the level of disuse (e.g., in muscle fibers/motor units not used during normal activity or in the case of immobilization due to injury), the greater the muscle atrophy and potential for lost strength.
Nonetheless, simple detraining is poorly understood relative to more extreme, medically relevant and better studied forms of disuse like spinal cord injury or immobilization (8). There are no guarantees as to how fit or unfit you may be when returning to training after a long layoff. Blending a bit of science with common sense may help you avoid thinking, “Well, there you go, Bozo—look at what you did to yourself” after being a bit too “gung ho” when getting back in the iron saddle.
References
- Cohen J, Stewart I (1994) The collapse of chaos: Discovering simplicity in a complex world. New York: Viking.
- Hakkinen K, Komi PV (1983) Electromyographic changes during strength training and detraining. Medicine & Science in Sports & Exercise 15(6):455–60.
- Houston ME, et al (1983) Muscle performance, morphology and metabolic capacity during strength training and detraining: A one leg model. Eur J Appl Physiol Occup Physiol 51(1):25–35.
- Shima N, et al (2002) Cross education of muscular strength during unilateral resistance training and detraining. Eur J Appl Physiol 86(4):287–94.
- Hakkinen K, et al (2000) Neuromuscular adaptation during prolonged strength training, detraining and re-strength-training in middle-aged and elderly people. Eur J Appl Physiol 83(1):51–62.
- Fiatarone MA, Evans WJ (1993) The etiology and reversibility of muscle dysfunction in the aged. J Gerontol 48(Spec No):77–83.
- Henwood TR, Taaffe DR (2008) Detraining and retraining in older adults following long-term muscle power or muscle strength specific training. J Gerontol A Biol Sci Med Sci 63(7):751–8.
- Urso ML (2009) Disuse atrophy of human skeletal muscle: cell signaling and potential interventions. Med Sci Sports Exerc 41(10):1860–8.
- Staron RS, et al (1991) Strength and skeletal muscle adaptations in heavy-resistance-trained women after detraining and retraining. J Appl Physiol 70(2):631–40.
- D’Antona G, et al (2006) Skeletal muscle hypertrophy and structure and function of skeletal muscle fibers in male bodybuilders. The Journal Of Physiology 570(Pt 3):611–27.
- Tesch PA (1988) Skeletal muscle adaptations consequent to long-term heavy resistance exercise. Med Sci Sports Exerc 20(S5):S132–4.
- Abernethy PJ, et al (1994) Acute and chronic response of skeletal muscle to resistance exercise. Sports Med 17(1):22–38.
- Mujika I, Padilla S (2001) Muscular characteristics of detraining in humans. Med Sci Sports Exerc 33(8):1297–303.
- MacDougall JD, et al (1980) Effects of strength training and immobilization on human muscle fibers. Eur J Appl Physiol Occup Physiol 43(1):25–34.
- MacDougall JD, et al (1977) Biochemical adaptation of human skeletal muscle to heavy resistance training and immobilization. J Appl Physiol Respir Environ Exerc Physiol 43(4):700–3.
- Staron RS, et al (1981) The effects of detraining on an elite power lifter. A case study. J Neurol Sci 51(2):247–57.
- Bruusgaard JC, et al (2010) Myonuclei acquired by overload exercise precede hypertrophy and are not lost on detraining. Proc Natl Acad Sci U S A 107(34):15111–6.
- Kadi F, et al (2004) The effects of heavy resistance training and detraining on satellite cells in human skeletal muscles. J Physiol 558(Pt 3):1005–12.
- Kadi F, et al (2005) The behavior of satellite cells in response to exercise: what have we learned from human studies? Pflugers Arch 451(2):319–27.
- McCarthy JJ, et al (2011) Effective fiber hypertrophy in satellite cell-depleted skeletal muscle. Development 138(17):3657–66.
- Sale DG, et al (1987) Voluntary strength and muscle characteristics in untrained men and women and male bodybuilders. Journal of Applied Physiology 62(5):1786–93.
- Antonio J, Gonyea WJ (1993) Skeletal muscle fiber hyperplasia. Medicine and Science in Sports and Exercise 25(12):1333–45.
- Staron RS, et al (1990) Muscle hypertrophy and fast fiber type conversions in heavy resistance-trained women. Eur J Appl Physiol Occup Physiol 60(1):71–9.
- Baar K (2010) Epigenetic control of skeletal muscle fiber type. Acta Physiol (Oxf) 199(4):477–87.
- Sousa-Victor P, et al (2011) Regulation of skeletal muscle stem cells through epigenetic mechanisms. Toxicol Mech Methods 21(4):334–42.
- McGee SL, et al (2009) Exercise-induced histone modifications in human skeletal muscle. J Physiol 587(Pt 24):5951–8.
- Matsuda R, et al (1983) Regenerating adult chicken skeletal muscle and satellite cell cultures express embryonic patterns of myosin and tropomyosin isoforms. Dev Biol 100(2):478–88.
- Berggren JR, et al (2005) Glucose uptake in muscle cell cultures from endurance-trained men. Med Sci Sports Exerc 37(4):579–84.
- Flann KL, et al (2011) Muscle damage and muscle remodeling: no pain, no gain? The Journal of Experimental Biology 214(Pt 4):674–9.
- Crameri RM, et al (2007) Myofibre damage in human skeletal muscle: effects of electrical stimulation versus voluntary contraction. J Physiol 583(Pt 1):365–80.
- Cheung K, et al (2003) Delayed onset muscle soreness : treatment strategies and performance factors. Sports Med 33(2):145–64.
- Craig JA, et al (1996) Lack of effect of transcutaneous electrical nerve stimulation upon experimentally induced delayed onset muscle soreness in humans. Pain 67(2–3):285–9.
- Babault N, et al (2011) Does electrical stimulation enhance post-exercise performance recovery? European Journal of Applied Physiology 111(10):2501–7.
- Baldwin AC, et al (2001) Nonsteroidal anti-inflammatory therapy after eccentric exercise in healthy older individuals. The Journals of Gerontology. Series A, Biological Sciences and Medical Sciences 56(8):M510–3.
- Schoenfeld BJ (2012) The Use of Nonsteroidal Anti-Inflammatory Drugs for Exercise-Induced Muscle Damage. Sports Medicine 42(12):1017–28.
- Bourgeois J, et al (1999) Naproxen does not alter indices of muscle damage in resistance-exercise trained men. Med Sci Sports Exerc 31(1):4–9.
- Baldwin Lanier A (2003) Use of nonsteroidal anti-inflammatory drugs following exercise-induced muscle injury. Sports Med 33(3):177–85.
- Nosaka K, et al (2001) How long does the protective effect on eccentric exercise-induced muscle damage last? Med Sci Sports Exerc 33(9):1490–5.
- Newton MJ, et al (2008) Comparison of responses to strenuous eccentric exercise of the elbow flexors between resistance-trained and untrained men. J Strength Cond Res 22(2):597–607.
- Nosaka K, Aoki MS (2011) Repeated bout effect: research update and future perspective. Brazilian Journal of Biomotricity 5(1).