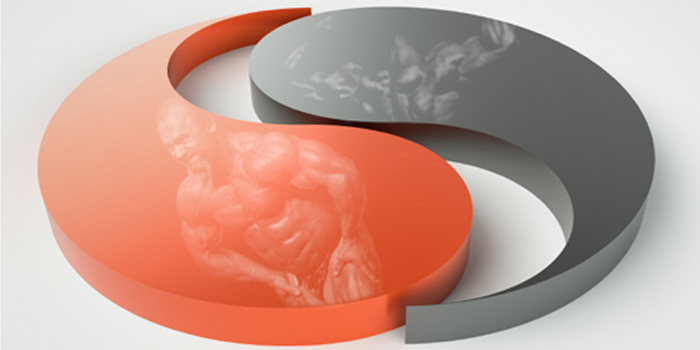
Much of the excitement of a high-level track and field meet is during the warm up, especially if you watch the sprinters readying themselves in the minutes before racing. It’s pretty obvious—they’re staying warm, priming muscle blood flow, staying limber for injury prevention, maintaining mental and neurological readiness with a ballistic warm-up protocol and even practicing key skill components of their events. These athletes are like drivers of high performance sports cars, revving engines, gripping steering wheels and keeping the clutch loose before tearing into impressive displays of athletic prowess cultivated through years, or even decades, of deliberate practice.
These pre-competition rituals are often highly individualistic, many acts meticulously conceptualized (and visualized) but some simply intuited and empirically derived. In other words, each athlete has her own style and flair in sequencing the events just before an impressive sprint, jump, or throw. Much of this may be psychological as well, but a particular physiological (and possibly neurological) mechanism could be in play. This phenomenon, called post-activation potentiation (PAP), has been known to muscle physiologists for decades, but its precise application still baffles sports scientists. My goal in this article is to make sure that PAP doesn’t baffle you.
A Warm Up and Then Some
The term "post-activation potentiation" sounds fancy, but it is just what is says. For a period of time after a muscle has been voluntarily activated (i.e. post-activation), the force it can produce is inherently increased (i.e. potentiated). The phenomenon was discovered in isolated muscle that had been stimulated electrically for a brief (one to two seconds) tetanic contraction. (In this case, the term "post-tetanic potentiation" (PTP) is used.) PTP manifests in a more forceful twitch when the muscle is given a singular impulse immediately (approximately five seconds later [1]) after the tetanus, but this effect fades over several minutes (2). Additionally, when stimulated to produce force at low frequencies (not rapidly enough to create steady and “fused” tetanic contractions), forces are higher due to PTP (3, 4). The potentiating effect is greater in type II muscle fibers (1), which are generally the faster, more powerful fibers and those that are more difficult to activate during maximal voluntary efforts (i.e. when exercising [5]).
Nature struck a beautiful balance in engineering mammalian skeletal muscle this way. The brain-to-muscle signal for producing force is mediated by elevated calcium levels inside the muscle cell. At the same time, the contraction also increases sensitivity to calcium via regulatory proteins (myosin light chain proteins) that become phosphorylated (2). Like a plane that could somehow taper its profile in flight to improve aerodynamics and reduce wind resistance, this is an effective strategy for offsetting fatigue in the fibers that have been activated at suboptimal (low) frequencies (3, 6, 7). Additionally, when muscle cells are modulating calcium levels to control force output, this “calcium handling” is energetically costly, especially in type II fibers (8). By making the muscle cell more sensitive to calcium, PTP means that less energy is needed for a given force level and fatigue is reduced.
All in the Muscle?
Not surprisingly, creating substantial PAP in the muscle tissue requires substantial voluntary effort. (Calcium is the signal for both contraction and potentiation.) High effort activity also increases sensitivity of the motor neurons to the signals sent from the brain, another mechanism for enhancing performance (3, 9–12). Some research has also suggested that potentiation during fatigue is regulated by an unknown factor (i.e., something in addition to myosin light chain phosphorylation [12, 13]). While this paints an even more interesting and exquisite picture of neuromuscular control, not knowing the relative contributions of contractile and neural factors and what they are exactly (11) makes manipulating PAP for improving performance all the more difficult.
What’s in the Black Box?
Several reviews have tackled the topic of PAP and performance (3, 11, 12, 14–16). The focus has been on improving power production via PAP (e.g. vertical jump height and sprinting). This research focus probably stems from early evidence showing improved rates of force production (1, 17, 18), greater acceleration and higher force at high contractile speed as a result of PAP (3, 11). (Increased sensitivity to intracellular calcium reduces the time to engage the contractile machinery as calcium levels increase, so force is produced more rapidly or more force is produced in these high power and high velocity scenarios.) Several basic findings of the research literature on this topic shine through. Generally, it seems that the more highly trained one is, the greater the possibility for PAP ergogenesis (19). This is probably due in part to greater resistance to fatigue (13). Training status (fatigue resistance) may also explain why stronger individuals show greater PAP effects (8). As noted above, greater type II fiber percentage seems to manifest in greater PAP effects, too (1, 20, 21). Although some studies have suggested such (17), gender does not seem to matter overall for PAP (11, 22).
On the other hand, much of the particulars of the practical application of PAP remain elusive. While tetanizing muscle (a complete contraction) reliably creates potentiation (3), it seems that moderate intensity efforts are best for PAP (22). Similarly, twitches of isolated muscle are most potentiated just after a brief tetanus (PTP), but resting longer than seven minutes seems best for PAP-induced performance enhancement (12, 15, 22, 23). Additionally, several studies have suggested a large variability in PAP effects across subjects, the type of contraction needed to elicit a PAP and the kind of performance that PAP can improve (12, 14, 16, 24). Indeed, there may even be non-responders when it comes to the phosphorylation of myosin light chain brought on by a “conditioning contraction” intended to produce PAP (25).
What Gives?
As you may have guessed, the overlap of fatigue with PAP may be partly to blame for the above confusion. The conditioning contraction (CC) creates fatigue that can negate the PAP effect. For instance, waiting just three minutes after the third of three sets of leg presses (90 percent of a one-rep maximum) is not long enough to potentiate maximal rate of force production when squatting (26). Although PAP protocols typically improve vertical jump performance and knee extension performance (3, 27), this is not always the case. A 10-second maximal effort may actually increase quadriceps twitch force just seconds later (when evoked electrically in humans) but create no performance benefit during high speed voluntary efforts (28) or vertical or drop jumps(12) simply because muscular fatigue is an issue. Muscle twitches (a primary identifier of a PAP or PTP effect) just aren’t the same as all-out dynamic, powerful movements. Because of this overlap between PAP and fatigue, it’s been hypothesized that one can open up two “windows of opportunity” to harness a PAP ergogenic effect. Which window opens depends on how the CC balances with the fatigue that it creates (3, 15):
- A brief, minimally fatiguing CC would result in an ergogenic effect shortly afterward (PAP outweighing fatigue).
- A more prolonged CC (such as during a workout) would elicit a ergogenic effect if fatigue dissipates faster than PAP over the course of several minutes.
The above (and possible neurological effects) has resulted in recommendations to perform plyometric efforts within 30 seconds of a CC as well as take up to 10 minutes between coupling a CC with a power/speed movement (16). (The CC and power/speed movement is called a complex pair, in this case [24, 29].) Several studies suggest a benefit of combining weight training and plyometrics in the same training regime (16, 24, 30), but only few (16, 24, 31), and none published in the western literature (32), have tested complex pair training in particular. (There is a study of complex pair training in early pubertal boys that didn't compare complex pair with more traditional training [33].) What’s sorely lacking here is evidence to help coaches fine tune whatever advantage can be had by PAP in the field, on the lifting platform or in the gym (24, 32, 34).
What’s This Got to Do with Bodybuilding?
Science doesn’t have all the answers. Oftentimes, as appears to be the case with making PAP ergogenic, scientific inquiry has simply generated more questions. Recent reviews have bluntly pointed out the equivocal nature of the research and that there are practical difficulties in coupling complex pairs during training (24, 32) if long rest intervals (about five minutes or longer) are needed (22). (Practically speaking, how long can a workout last?)
Putting the above aside, bodybuilding is not a power sport. (Some would say that it isn't a sport at all.) The purpose of training is to create a hypertrophic remodeling stimulus, be it via muscular loading (35), metabolic stress (36) and/or damage and inflammation (37). Thus, in the context of bodybuilding, PAP may very well be able to increase muscle loading simply by reducing fatigue (3). In other words, if one can do more reps with a given weight, this means a more powerful training stimulus and growth response (38, 39).
During the course of a fatiguing set, the frequency at which motor units are activated decreases, a phenomenon known as “muscle wisdom.” (It’s actually the nervous system “wisely” matching firing rates to what’s needed to minimize fatigue and optimize force [40].) Although difficult to test during a resistance exercise bout (41, 42), the well studied “central fatigue” hypothesis (43) suggests that, despite maximal effort, some high threshold motor units simply won’t be activated continuously or at optimal frequencies (43–45). So a PAP effect that sensitizes muscle cells to calcium levels and counters “low frequency” fatigue during those last grueling reps (when your nervous system is struggling to produce ideal motor unit firing rates) may help you squeeze out an extra repetition or two (6). PAP may improve the training stimulus in this way. (Unfortunately, the ergogenic effects of coupling conditioning contractions with one or more normal resistance exercise straight sets has not been studied directly to my knowledge, so this is my speculation and that of others [6].)
Remember that PAP may have a neurological basis. A recent meta-analysis suggests that maximal effort lower loads (60–84 percent of a one-repetition maximum) are more effective as conditioning contractions than heavier loads (22). A study using chains set up to reduce load at the bottom of a bench press by 40 percent demonstrated improved power (compared to continuous “normal” loading with a regular barbell) during maximal effort three-rep sets (75 percent one-rep maximum). The authors suggested that this could be due to a “within repetition” PAP effect (46, 47). In other words, the heavier loading of each repetition near lockout potentiated (neurologically) the subjects’ concentric pressing power and repetition speed when at the start of the press (off the chest). In general, faster repetition speed results in more repetitions for a given load (48), so using chains or bands to facilitate concentric pressing power could enhance the muscle growth stimulus. Naturally, this would require greater muscular loading under fatiguing conditions when using bands or chains (an ergogenic effect of PAP). (In lieu of an ergogenic potentiating effect, performing more repetitions simply because the bar is being unloaded is probably less likely to translate into greater growth any more so than simply using a lighter load in general.)
Have Bodybuilders Been Using PAP All Along?
Alternating sets for two muscle groups (e.g. between rowing and pressing movements) is a technique that bodybuilders have used for decades and it might be one way to dissipate fatigue and harness a PAP effect. Bands and chains are now being used by quite a few bodybuilders (ushered in largely by John Meadows) and, as noted above, may also be exploiting a “within repetition” PAP effect. Combining these two approaches by successively training opposing muscle groups and using bands or chains may be a novel way to utilize PAP without wasting precious gym time waiting for fatigue to dissipate after a conditioning contraction. A simple example (that wouldn't require more than one “station” in the gym) might be to use 60–85 percent one-rep maximum loads (roughly optimal for PAP [22, 46, 47, 49]) and alternate between a flat bench with chains (or bands) or a bent-over row with chains (or bands).
Three minutes of rest between each set would end up giving approximately seven minutes of recovery for each muscle group/movement, which the data to date suggest works best for PAP (22, 50). This rest interval would give enough time for training partners to use the same bar and adjust the weight for both lifts as needed.
Note that there is marked variability in PAP effects across individuals and muscles involved (12, 14, 16, 24). Some in-the-gym tinkering (and careful log book evaluation) might be needed to fine tune and evaluate whether this kind of approach is advantageous. Hands-on use of PAP to improve performance is only dimly lit at this point (at least in the western scientific literature). There may simply be little practical means of exploiting this fascinating neuromuscular phenomenon. Nonetheless, I hope that you now have a better understanding of what post-activation potentiation is and that the next time you hear or read this fancy term, you are filled with informed curiosity (or criticism) about the topic at hand rather than disinterest and/or confusion.
References
- O'Leary DD, et al (1997) Post-tetanic potentiation of human dorsiflexors. J Appl Physiol (1985) 83(6):2131–8.
- Kamm KE, Stull JT (2011) Signaling to myosin regulatory light chain in sarcomeres. J Biol Chem 286(12):9941–7.
- Sale DG (2002) Post-activation potentiation: role in human performance. Exerc Sport Sci Rev 30(3):138–43.
- Abbate F, et al (2000) Effects of high-frequency initial pulses and post-tetanic potentiation on power output of skeletal muscle. J Appl Physiol (1985) 88(1):35–40.
- Enoka RM (2005) Central modulation of motor unit activity. Med Sci Sports Exerc 37(12):2111–2.
- Sale D (2004) Post-activation potentiation: role in performance. Br J Sports Med 38(4):386–7.
- Rassier DE, Macintosh BR (2000) Coexistence of potentiation and fatigue in skeletal muscle. Braz J Med Biol Res 33(5):499–508.
- Szentesi P, et al (2001), ATP utilization for calcium uptake and force production in different types of human skeletal muscle fibres. J Physiol 531(Pt 2):393–403.
- Aagaard P (2003) Training-induced changes in neural function. Exerc Sport Sci Rev 31(2):61–7.
- Aagaard P, et al (2002) Neural adaptation to resistance training: changes in evoked V-wave and H-reflex responses. J Appl Physiol (1985) 92(6):2309–18.
- Xenofondos A, et al (2010) Post-activation potentiation: factors affecting it and the effect on performance. Citius Altius Fortius 28(3):32–8.
- Güllich A, Schmidtbleicher D (1996) MVC-induced short-term potentiation of explosive force. New Studies in Athletics 11:67–84.
- Chiu LZ, et al (2003) Post-activation potentiation response in athletic and recreationally trained individuals. J Strength Cond Res 17(4):671–7.
- Comyns TM, et al (2006) The optimal complex training rest interval for athletes from anaerobic sports. J Strength Cond Res 20(3):471–6.
- Tillin NA, Bishop D (2009) Factors modulating post-activation potentiation and its effect on performance of subsequent explosive activities. Sports Med 39(2):147–66.
- Ebben WP, Watts PB (1998) A review of combined weight training and plyometric training modes: Complex training. Strength & Conditioning Journal 20(5):18–27.
- O'Leary DD, et al (1998) Influence of gender on post-tetanic potentiation in human dorsiflexors. Can J Physiol Pharmacol 76(7–8):772–9.
- Vandenboom R, et al (1993) Threshold for force potentiation associated with skeletal myosin phosphorylation. Am J Physiol 265(6 Pt 1):C1456–62.
- Rixon KP, et al (2007) Influence of type of muscle contraction, gender, and lifting experience on post-activation potentiation performance. J Strength Cond Res 21(2):500–5.
- Hamada T, et al (2000) Post-activation potentiation, fiber type, and twitch contraction time in human knee extensor muscles. J Appl Physiol (1985) 88(6):2131–7.
- Hamada T, et al (2003) Interaction of fibre type, potentiation and fatigue in human knee extensor muscles. Acta Physiol Scand 178(2):165–73.
- Wilson JM, et al (2013) Meta-analysis of post-activation potentiation and power: effects of conditioning activity, volume, gender, rest periods, and training status. J Strength Cond Res 27(3):854–9.
- Gouvea AL, et al (2013) The effects of rest intervals on jumping performance: A meta-analysis on post-activation potentiation studies. J Sports Sci 31(5): 459–67.
- Robbins DW (2005) Post-activation potentiation and its practical applicability: a brief review. J Strength Cond Res 19(2):453–8.
- Smith JC, Fry AC (2007) Effects of a Ten-Second Maximum Voluntary Contraction on Regulatory Myosin Light-Chain Phosphorylation and Dynamic Performance Measures. The Journal of Strength & Conditioning Research 21(1):73–6.
- Bazett-Jones DM, et al (2005) Effect of potentiation and stretching on maximal force, rate of force development, and range of motion. The Journal of Strength & Conditioning Research 19(2):421–26.
- French DN, et al (2003) Changes in dynamic exercise performance following a sequence of preconditioning isometric muscle actions. The Journal of Strength & Conditioning Research 17(4):678–85.
- Gossen ER, Sale DG (2000) Effect of post-activation potentiation on dynamic knee extension performance. Eur J Appl Physiol 83(6):524–30.
- Chu DA (1996) Explosive power & strength: Complex training for maximum results. Human Kinetics: Champaign, IL.
- Adams K, et al (1992) The effect of six weeks of squat, plyometric and squat-plyometric training on power production. The Journal of Strength & Conditioning Research 6(1):36–41.
- Verkhoshansky Y, Tatyan V (1973) Speed-strength preparation of future champions. Legkaya Atleika 2:12–13.
- Docherty D, Hodgson MJ (2007) The application of post-activation potentiation to elite sport. International Journal of Sports Physiology & Performance 2(4).
- Ingle L, et al (2006) The effect of a complex training and detraining program on selected strength and power variables in early pubertal boys. J Sports Sci 24(9):987–97.
- Hodgson M, et al (2005) Post-activation potentiation: underlying physiology and implications for motor performance. Sports Med 35(7):585–95.
- Schoenfeld BJ (2010) The mechanisms of muscle hypertrophy and their application to resistance training. Journal of strength and conditioning research/National Strength & Conditioning Association 24(10):2857–72.
- Schoenfeld BJ (2013) Potential Mechanisms for a Role of Metabolic Stress in Hypertrophic Adaptations to Resistance Training. Sports medicine 43(3):179–94.
- Schoenfeld BJ (2012) The Use of Nonsteroidal Anti-Inflammatory Drugs for Exercise-Induced Muscle Damage. Sports Medicine 42(12):1017–28.
- Burd NA, et al (2012) Muscle time under tension during resistance exercise stimulates differential muscle protein sub-fractional synthetic responses in men. J Physiol 590(Pt 2):351–62.
- Hornberger TA (2011) Mechanotransduction and the regulation of mTORC1 signaling in skeletal muscle. The International Journal of Biochemistry & Cell Biology 43(9):1267–76.
- Gandevia SC (2001) Spinal and supraspinal factors in human muscle fatigue. Physiol Rev 81(4):1725–89.
- Farina D, et al (2004) The extraction of neural strategies from the surface EMG. J Appl Physiol (1985) 96(4):1486–95.
- Enoka RM (2012) Muscle fatigue--from motor units to clinical symptoms. J Biomech 45(3):427–33.
- Tanaka M, Watanabe Y (2012) Supraspinal regulation of physical fatigue. Neurosci Biobehav Rev 36(1):727–34.
- Gandevia SC, et al (1996) Supraspinal factors in human muscle fatigue: evidence for suboptimal output from the motor cortex. J Physiol 490(Pt 2):529–36.
- Grimby L, et al (1981) The fatigue and voluntary discharge properties of single motor units in man. J Physiol 316:545–54.
- Baker D (2003) Acute effect of alternating heavy and light resistances on power output during upper-body complex power training. J Strength Cond Res 17(3):493–7.
- Baker DG, Newton RU (2009) Effect of kinetically altering a repetition via the use of chain resistance on velocity during the bench press. J Strength Cond Res 23(7):1941–6.
- Sakamoto A, Sinclair PJ (2006) Effect of movement velocity on the relationship between training load and the number of repetitions of bench press. J Strength Cond Res 20(3):523–7.
- Chatzopoulos DE, et al (2007) Post-activation potentiation effects after heavy resistance exercise on running speed. The Journal of Strength & Conditioning Research 21(4):1278–81.
- de Assis Ferreira SL, et al (2012) Post-activation Potentiation: Effect of Various Recovery Intervals on Bench Press Power Performance. The Journal of Strength & Conditioning Research 26(3):739–44.
Thanks for the article!