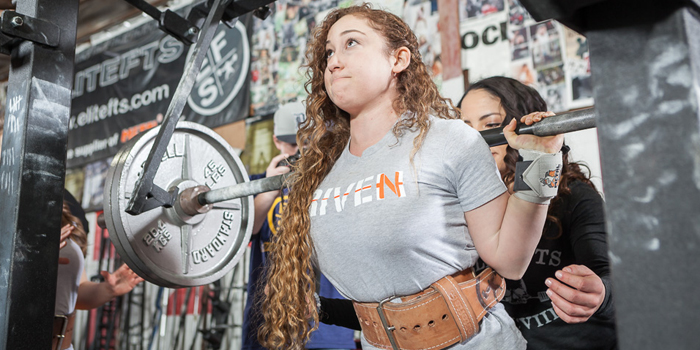
The idea of infinite hypertrophy is somewhat of a pipe dream, chased after by the multi-billion dollar sports supplement industry and the athletes devoted to figuring out how to achieve it. We know that the structural capabilities of our tendons, bones, and joints limit their abilities to support never ending muscle hypertrophy; however, many still search for ways to manipulate the intrinsic muscle factors that limit muscle growth.
The supplement industry will tell you that there are magic pills and powders that help you evade hypertrophy plateaus. I’m not here to say that those things can’t help you gain muscle, because they can. Sometimes though, they crash your wallet in trial and error process of figuring out which supplements work for you. While there are many things that limit muscle growth, I’m here to talk to you about just one. This “supplement“ is free, easily accessible, and critical to acquiring muscle mass.
The “supplement” I’m talking about is oxygen, and it is essentially everything when it comes to continual muscle growth. Now, for those of you thinking, “Jenn, we all need oxygen,” I agree. But, I’m here to talk to you about why oxygen is critical for hypertrophy (muscle fiber growth) and how you can maximize this free magical substance to help keep you muscles growing.
Before we get going, we’re going to backtrack. We are going to cover some basics about muscle contractions and hypertrophy, as well as, review fiber types and the role of oxygen in muscle growth. Then, once we’re all on the same page, we will go over simple ways to tackle this limitation in your quest for infinite hypertrophy.
Hypertrophic Growth and Energy
We could talk all day about what supplements work and why they work when it comes to skeletal muscle hypertrophy, but the truth is, none of that matters if we don’t understand hypertrophy in general. There are three types of growth going on in muscle in your lifespan: incremental growth, reparative growth, and hypertrophic growth. To make hypertrophic growth happen, we train and induce stressors that tell the muscle to increase in shape, functionality, density, and mass as a way to meet the demands that training imposes. Thus, as you increase the stimuli to the muscle, and increase the functional demands, muscle adapts by increasing contractile, structural, and enzymatic proteins.
Many people will tell you that hormones and nutritional support are what drive hypertrophic growth, but the truth is, it’s relatively independent from those factors. Actually, that’s one of the things that make hypertrophic growth different from incremental and reparative growth. So what does that mean? Well, it means that you can have hypertrophic growth in the absence of exogenous nutrient intake and hormones, as long as your body has the ability to reallocate existing energy supplies to the process. Sorry, fan of bulking, I’m basically telling you hypertrophy can happen without a massive amount of calories1. You see, each time you lift weights, mechanical tension is applied to the membrane of cells within your muscles. This can activate the same anabolic pathways that hormones and nutrients do. Thus, mechanical tension alone can induce protein synthesis2. Don’t misunderstand me, nutrients and hormones obviously help the growth process and increase its magnitude. But, it is the metabolic (O2 supply) and mechanical (training induced overload) demands that really run the muscle growth show.
Oxygen’s Role in Making Your Muscles Move
When you lift weights and train, you do hundreds and thousands of repetitions in an attempt to induce sufficient damage to your muscles so that they can repair and come back bigger and better than ever. Let’s zoom into what’s happening during each repetition. First, your brain sends signals called action potentials to your muscles telling them to contract. As muscles contract, or shorten, contractile units within each myofibril shorten. These contractile units are called sarcomeres, and they run in a stacked pattern throughout muscle tissue3. Within each sarcomere, there are contractile proteins called myosin and actin that use ATP and calcium to promote sarcomere shortening. We actually call this the sliding filament theory. Thus, we need both calcium and adenosine triphosphate (ATP) to keep muscle contractions going.
During your training session, muscle glycogen and glucose are continuously broken down to make ATP. Fat, or more specifically fatty acids, can also be broken down to yield ATP, but this tends to take a lot longer. To get ATP made in large quantities, your muscles have these structures called mitochondria. Mitochondria are the powerhouses of cells. We call mitochondria the powerhouses because they generate energy for the cell, in the form of ATP. Through the transportation of oxygen to working muscles via its diffusion from the capillaries, your mitochondria use O2 to convert macronutrients (carbohydrates, fats, and/or proteins) into ATP. We call that process aerobic metabolism.
RECENT: Failure: A Way to Train Your Fortitude
Since ATP drives the muscle contraction, you need O2 to make ATP when you rely on aerobic metabolism. O2 in the muscle can become limited if contraction intensities become to great or if the demand of O2 exceeds its availability. Thus, ATP levels become depleted and your anaerobic energy supplies become depleted, fatigue sets in. Don’t get me wrong, your anaerobic metabolism is very important and working hard to generate ATP while you lift. However, in those long, high rep sets, you also heavily rely on your aerobic metabolism because your anaerobic system gets depleted first. Furthermore, it’s your aerobic system that promotes your recovery and generation of new anaerobic energy supplies for the next set. Thus, the ability for you to get oxygen into your muscle following the set is a huge mediator of the intensity you can apply to your next set.
Muscle Fiber Types and Hypertrophy
Hypertrophic growth means that you are increasing in the size of muscle fibers. Muscle fibers set out to accomplish the tasks that you ask of them during exercise, and as a result, fibers adapt. Fiber can be classified into different types: Type I, IIA, IIX, and IIB (sometimes you will see IIX and IIB written as IIX/B). These fibers types are classified by the myosin motor protein isoform they express (MHC I, IIA, IIX) or co-express (MHC I/IIA, MHC IIA/IIX, MHC I/IIA/IIX). Myosin, which we mentioned earlier, is one of those contractile proteins that drives the shortening of your muscles as they contract3. Additionally, myosin isoforms dictate the speed and durations of contractions. Depending on the demand you impose on the body, it is possible to induce a fiber type shift so that your muscles can execute the movements you ask of them at a minimal metabolic cost4. Thus, you can imagine that there is an internal muscle feedback loop which links information about the contractile demand, the ATP needed to supply that demand, and the amount hypertrophic growth that occurs.
Your Type I fibers are known as the slow-twitch fibers. This means they have slow contraction rates. They also have a lot of mitochondria. Thus, your Type I fibers, can generate a lot of ATP using aerobic metabolism. Because Type I fibers can make a lot of ATP, they are more resistant to fatigue than Type II fibers. Thus, in the presence of sufficient oxygen and exogenous/endogenous macronutrient supply, these fibers can keep cranking out energy. With a lot of cardiovascular activity, you can shift the metabolic activity within the muscle by acquiring more oxidative enzymes. This can promote the predominance of Type I fibers. Because of this, you might hear people say, “don’t do too much cardio if you want to be a fast, explosive, lifter.” However, please note, fiber type shifting overnight and takes a large amount of training to induce. One run is not going to make you a Type I predominate athlete.
Your Type II (IIX and IIB) fibers are both your easily fatiguable, glycolytic, fast-twitch fibers. This makes sense considering that they have less mitochondria to pump our energy. Thus, to be explosive, they rely more heavily on your anaerobic metabolism (without O2) to generate energy when working (not at baseline). Type IIX and IIA are not exactly the same though, as Type IIAs are your glycolytic-oxidative fast-twitch fibers. Thus, they have benefits of being glycolytic, powerful, and explosive, while still being somewhat fatigue resistant and oxidative. With the exception of one study, most of the research has shown that bodybuilders have predominantly MHC IIA fibers.
Additionally, they have very few, to no, MHC IIB/X fibers5-7. Strength training has been show to promote a unilateral shift from IIX to IIA fibers8-11 and explosive activities like sprinting are reported to elicit a bidirectional shift where MHC I and IIX both shift towards IIA12. Thus, bodybuilders, or those in search of infinite hypertrophy actually have a greater proportion of IIA fibers, and not IIX or I/IIA/IIX fibers compared to normal individuals who exercise. If you’re wondering why, that is a good question. But think about it. As you train, your muscles are working. In the heavy sets, you are predominately pounding your anaerobic metabolism to supply you with energy to move the weight and during rest periods, your aerobic metabolism is working hard to replenish your depleted energy stores. Additionally, those high rep failure sets really push blood and oxygen to the muscle, which helps promote the generation of a lot of energy when you have sufficient mitochondrial content. Thus, shifting to a IIA fiber, AKA adding more mitochondria while keeping the fast fiber phenotype, is really just your muscles adapting to the demands you’re imposing.
MORE: The Best Supplement Ever?
Not to confuse you, but Type IIX has been known to be the largest type of fiber. These fibers break down ATP the fastest and have the least amount of mitochondria. I know, this seems to make almost makes no sense at first, because I’m essentially tell you the fibers which have the potential to be the biggest have the least ATP powerhouses? But think about where you find the people with the most Type IIX fibers. These are often the people who have become immobilized or inactive. Inactivity alone leads to the conversion of muscle fibers into IIX. That, of course, should be surprising to some, as many people will tell you it is the Type II fibers that make you explosive. However, elderly people who have more Type IIX fibers also have lower maximal oxygen consumption rates (vO2max). These people are usually weaker and experience the opposite of hypertrophy, atrophy. Elderly people are often immobile and as they age, the oxidative capacity of their working muscles declines. We actually know that mitochondrial biogenesis (making new mitochondria) declines occurs with aging13 and concurrently elderly people experience a shift to more type IIX fibers. Thus, their fibers become more glycolytic, less oxidative, and make less ATP because they have less mitochondria. It all starts to make sense when you consider that glycolytic fibers have longer diffusion distances, and as a result, a larger fiber is required to supply adequate amounts of O2. With less O2 being delivered, the muscle responds by reducing its metabolic demands and metabolic costs of normal function by converting to a IIX fiber14. At that same time, muscle that isn’t being used becomes lost. Thus, in the absence of directing blood flow to the muscle and remaining active, the muscle gets rid of its fibers that consume higher basal levels of O2, and allows those with less O2 demands to predominate15,16. Keeping this train of thought in mind, it is almost as if we can make the observation that oxygen availability, and the oxidative capacity of a muscle, are critically important in the regulation of muscle growth.
Oxygen and Muscle Growth
As your muscles grow in response to training, existing capillaries that supply O2 get pushed away, thus increasing the diffusion distance, or distance of a muscle that a capillary must oxygenate. Capillary neoformation occurs with muscle growth to attempt to increase the O2 available to fuel that muscle, but not to the extent that your muscle is hypertrophying. This mismatch results in a decreased O2 supply and capillary density in larger muscles17,18, thus providing a checkpoint to unchecked muscle growth.
A state without oxygen is known as anaerobic, but this anaerobic environment also hypoxic, because by definition, a hypoxic state is one where there is not an adequate oxygen supply. When your muscle is faced with an anaerobic environment like resistance training, it adapts by upregulating the expression of genes that provide more blood and oxygen to the muscle. Specifically, the mitochondria have a protein within them that senses the training induced hypoxic environment. This then causes a series of events that results in an increase in genes and proteins, like erythropoietin (EPO)19,20 and vascular endothelial growth factor (VEGF)21, which promote getting more O2 into the muscle.
So perhaps you are thinking, why would muscles have a built in mechanism to sense low energy and why would they respond to that signal by increasing proteins that promote the increase in O2 supply? Well, it’s because when you demand that your muscles give you hypertrophy, they respond by making sure you have the necessary things for hypertrophy to occur. And, O2 just happens to be one of those necessary things.
Now, I’m sure there are some of you sitting there thinking, “Jenn, cardio builds oxidative capacity, and cardio kills muscle mass gains. “ I’m here to tell you though that you need O2 for your muscles to continue to growth, and without a sufficient oxidative capacity, your muscles can’t keep growing. Don’t believe me? Well, mammalian target of rapamycin (mTOR), the thing most people think about when we think about activation of anabolic pathways on the molecular level, is actually involved in controlling oxygen consumption and genes that regulate mitochondrial biogenesis (the formation of new mitochondria)22. Thus mTOR’s ability to activate metabolic genes could be a way to view that the muscle is always looking for ways to increase its energy levels to support its anabolic growth. Additionally, without sufficient O2, we activate proteins that increase myofibriliar degradation, thus limiting muscle fiber size. There are physiological mechanisms in place to make sure muscle can grow in an environment of sufficient O2. When that environment is no longer supplied with sufficient oxygen though, muscle growth is halted. When this halt on growth is implement, it promotes sufficient oxygenation of the muscle, and thus more energy. Remember what I said earlier: muscle doesn’t need nutrients to hypertrophy if it has the means to do mobilize internal energy22. And, those cellular energy levels really rely on the presence of O2, since it is aerobic, not anaerobic metabolism, which predominately supplies the basal day-to-day energy needs of skeletal muscle.
What to Do
By now, hopefully I’ve convinced you that your muscles need O2 to keep growing. I won’t lie, though, there is data out there showing that cardio blunts hypertrophy23,24. Some people hypothesize that cardio-induced catabolic pathways inhibiting anabolic ones25, thus halting training gains. Others believe hypertrophy is halted by cardio because training volume becomes greater than your ability to recover. What side of the fence I sit on in regards to that debate is a topic for another time. However, please note that cardiovascular activity can help you on your quest for hypertrophic gains. To do this, we need to think about ways to increase the oxidative capacity of your muscles, without inhibiting your explosiveness or recovery. So, without further ado, here are my suggestions (which have no scientific support because there is limited research on this topic, to my knowledge).
- Walking on the treadmill. Walking will increase your aerobic capacity, because it is a form or aerobic cardiovascular exercise. At a low intensity, it should not be enough of a stressor to cause your muscles to become masses of Type Is. Also, at a low intensity it should help balance an overactive sympathetic response to resistance training, since it is a parasympathetic stimulus. Thus, with low intensity walking, you can prevent overtraining and enhance the oxidative capacity of your muscles. This is what we call a double win.
- Assistance exercise with high rep band work. This type of concentric-only exercise, at a low intensity, will push blood flow and oxygen into the muscles. Pretty much, it’s giving your muscles the things they need to grow. Also, it shouldn't add more work to recover from, since concentric movements, unlike eccentric or triphasic movements, are not as hard to recover from. Actually, a lot of people use sled work (concentric only) to help improve their recovery.
- Short rest intervals between sets. Short rest intervals, like on dynamic work or supersets, will increase your heart rate and thus increase stress to the circulatory and respiratory systems. Short rest intervals actually help train your cardiovascular fitness, believe it or not. Thus, when you use submaximal loads and keep the rest short between sets, you are doing cardio, to an extent. How great is that? Also, since it is not adding more training volume, it shouldn’t hinder your ability to recover from your training session.
Now, get out there and get jacked and oxygenated!
References
1. Goldberg, A.L., Etlinger, J.D., Goldspink, D.F. & Jablecki, C. Mechanism of work-induced hypertrophy of skeletal muscle. Medicine and science in sports 7, 185-198 (1975).
2. Borer, K.T. Exercise endocrinology, (Human Kinetics, Champaign, IL, 2003).
3. Smith, D.A. & Sicilia, S. The theory of sliding filament models for muscle contraction. I. The two-state model. Journal of theoretical biology 127, 1-30 (1987).
4. Conley, K.E. Cellular energetics during exercise. Advances in veterinary science and comparative medicine 38A, 1-39 (1994).
5. Mishra, P., Varuzhanyan, G., Pham, A.H. & Chan, D.C. Mitochondrial Dynamics Is a Distinguishing Feature of Skeletal Muscle Fiber Types and Regulates Organellar Compartmentalization. Cell metabolism 22, 1033-1044 (2015).
6. Kesidis, N., et al. Myosin heavy chain isoform distribution in single fibres of bodybuilders. European journal of applied physiology 103, 579-583 (2008).
7. Klitgaard, H., Zhou, M. & Richter, E.A. Myosin heavy chain composition of single fibres from m. biceps brachii of male body builders. Acta physiologica Scandinavica 140, 175-180 (1990).
8. Adams, G.R., Hather, B.M., Baldwin, K.M. & Dudley, G.A. Skeletal muscle myosin heavy chain composition and resistance training. Journal of applied physiology 74, 911-915 (1993).
9. Staron, R.S., et al. Muscle hypertrophy and fast fiber type conversions in heavy resistance-trained women. European journal of applied physiology and occupational physiology 60, 71-79 (1990).
10. Hather, B.M., Tesch, P.A., Buchanan, P. & Dudley, G.A. Influence of eccentric actions on skeletal muscle adaptations to resistance training. Acta physiologica Scandinavica 143, 177-185 (1991).
11. Staron, R.S., et al. Assessment of skeletal muscle damage in successive biopsies from strength-trained and untrained men and women. European journal of applied physiology and occupational physiology 65, 258-264 (1992).
12. Andersen, J.L., Klitgaard, H. & Saltin, B. Myosin heavy chain isoforms in single fibres from m. vastus lateralis of sprinters: influence of training. Acta physiologica Scandinavica 151, 135-142 (1994).
13. Sahin, E. & DePinho, R.A. Axis of ageing: telomeres, p53 and mitochondria. Nature reviews. Molecular cell biology 13, 397-404 (2012).
14. Jimenez, A.G., Dasika, S.K., Locke, B.R. & Kinsey, S.T. An evaluation of muscle maintenance costs during fiber hypertrophy in the lobster Homarus americanus: are larger muscle fibers cheaper to maintain? The Journal of experimental biology 214, 3688-3697 (2011).
15. Morgan, M.J. & Loughna, P.T. Work overload induced changes in fast and slow skeletal muscle myosin heavy chain gene expression. FEBS letters 255, 427-430 (1989).
16. Swynghedauw, B. Developmental and functional adaptation of contractile proteins in cardiac and skeletal muscles. Physiological reviews 66, 710-771 (1986).
17. Plyley, M.J., Olmstead, B.J. & Noble, E.G. Time course of changes in capillarization in hypertrophied rat plantaris muscle. Journal of applied physiology 84, 902-907 (1998).
18. Degens, H., Turek, Z., Hoofd, L.J., Van't Hof, M.A. & Binkhorst, R.A. The relationship between capillarisation and fibre types during compensatory hypertrophy of the plantaris muscle in the rat. Journal of anatomy 180 ( Pt 3), 455-463 (1992).
19. Scoppetta, C. & Grassi, F. Erythropoietin: a new tool for muscle disorders? Medical hypotheses 63, 73-75 (2004).
20. Kuriyama, S., et al. [Effect of human recombinant erythropoietin (Epo) on cellular Ca2+, Na+, and K+ regulation of vascular smooth muscle cells grown in vitro--a new insight into the pathogenesis of Epo induced hypertension]. Nihon Jinzo Gakkai shi 35, 671-678 (1993).
21. Wagner, P.D. The critical role of VEGF in skeletal muscle angiogenesis and blood flow. Biochemical Society transactions 39, 1556-1559 (2011).
22. Cunningham, J.T., et al. mTOR controls mitochondrial oxidative function through a YY1-PGC-1alpha transcriptional complex. Nature 450, 736-740 (2007).
23. Kraemer, W.J., et al. Compatibility of high-intensity strength and endurance training on hormonal and skeletal muscle adaptations. Journal of applied physiology 78, 976-989 (1995).
24. Bell, G.J., Syrotuik, D., Martin, T.P., Burnham, R. & Quinney, H.A. Effect of concurrent strength and endurance training on skeletal muscle properties and hormone concentrations in humans. European journal of applied physiology 81, 418-427 (2000).
25. Hickson, R.C. Interference of strength development by simultaneously training for strength and endurance. European journal of applied physiology and occupational physiology 45, 255-263 (1980).
I'm glad you asked about AMPK. I kind of intentionally kept it out of the article because I didn't want to get too molecular, but since you asked, the interaction between mTOR and AMPK is more complicated than people realize. So it goes like this: Increasing the AMP: ATP level (like with exercise) activates the gamma subunit of AMPK, the alpha subunit gets activated by things like muscle contractions/calcium calmodulin, a protein named LKB1, etc and the beta subunit gets activated by things like carbohydrate depletion. When AMPK is activated, it activates another protein named PGC-1a, which we call the master regulator of mitochondrial biogenesis. Now there is this protein called YY1. High levels of YY1 promote unchecked cell proliferation, as microRNA 29 transcription is block. This is important because mir29 is important to degrading YY1 mRNA, which decreases YY1, and allows myoblasts (muscle cells on differentiation path) to fuse. Following exercise induced injury and repair it is really important for myoblasts to fuse with/into myotubes, and not just undergo continual proliferation without fusion. Now, get this, mTOR and YY1 are actually recruited to the promoter of PGC1a and regulate the expression of this gene that controls mitochondrial biogenesis in a very complicated way. Knowndown of YY1 reduces mitochondrial gene expression and respiration, and inhibition of mTOR reduces PGC1a gene expression. Now, mTOR interacts with YY1, and it is believed that mTOR, YY1, and PGC-1a are recruited in a complex which binds the promoter of mitochondrial genes and regulates their expression.When mTOR is inhibited (and can't interact with YY1), the mTOR-YY1-PGC complex disassembles (YY1 no longer interacts with PGC1a) and the expression of mitochondrial genes is suppressed. So how does this relate? Well, it relates in that exercise, the repair after, and the supercompensation that leads to muscle growth is complicated. We have to carefully interpret data related to AMPK and mTOR because the timing the samples were collected, and how they are prepared, is really everything in terms of the results we get and what they mean. Also, just because protein expression of one is down/up or mRNA is down/up, this doesn't mean those proteins or genes are doing what we would expect. Further mechanistic studies looking at protein-dna, protein-protein, and protein-mRNA interactions are really needed to understand the complexity of the overlap and opposition in the AMPK and mTOR pathways. People have long thought the two oppose each other in terms of training, when in reality, they both can induce PGC-1a expression. So in terms of your question, "does one over-ride the other when you do cardio and then lift," the only way I can answer that for you is to tell you until I see some mechanistic studies looking at this, it's hard to say. A lot of these proteins and their expression really fluctuate, as do their interactions and functions. In terms of AMPK and this article though, I can tell you that both lifting and aerobic exercise will activate AMPK. Let me know if you have any other questions of if you want further explanations! Jenn (sorry for typos, didn't have a ton of time to proof this, at work!)