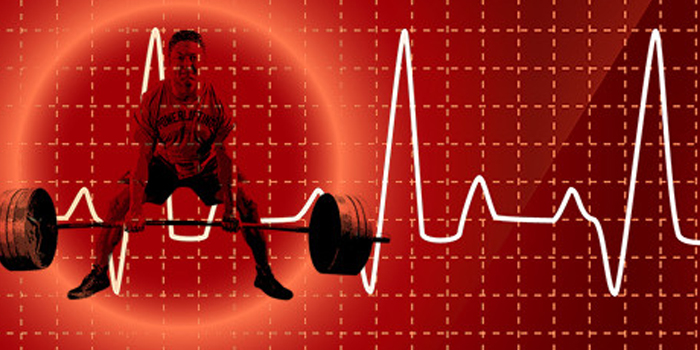
In part one of this series, we thoroughly examined the real truth about energy systems, their classification, the specifics of each energy system and the correct terminology used as well as how each energy system is interconnected and contributes to one another for energy provisions. One of the more confusing aspects is the role of fuel use during training, and even during recovery, and how intensity has large implications for these energy systems. Many are discussing and even advocating basic programming ideas based on these systems without ever really thinking and knowing about how they actually work. This article will help set the record straight and examine the real truth about energy systems.
The way that the body converts food to fuel is reliant on several different energy pathways, which was thoroughly discussed in part one. When training, your body will use the carbs stored in your liver and muscle as fuel. We know this as glycogen stores (see table 1). These stored carbs originate from the foods you eat. However, do we always train with our carb stores full? Not always. How does that affect performance? Well, just like many things training, nutrition and physiology related, it depends. Currently, a variety of nutritional methods are being used by numerous lifters and trainees to augment carb stores in favor of using fat. These include ketogenic diets (24, 27, 41), lower carb diets (27, 35–39) and carb back loading. However, regardless of what type of method you use, understand that different fuel sources and training intensity won't only dictate performance but how that performance can be maximized throughout any given training session and competition.
Table 1: Sites and amounts of stored carbs in rested, moderately active 70-kg man with 40 percent of body mass as skeletal muscle.
**Indicates range
As indicated, the values coincide with a 70-kg (or 154-pound) man, probably 160 pounds soaking wet with a moderate amount of lean muscle mass. So you can imagine how many grams of stored carbs and calories may be available for someone who is 230 pounds, 260 pounds or 300 pounds and has a lot of lean muscle tissue. The same goes for lean and strong women, too, though they have less available storage compared to their counterparts.
Although there can exist an astronomical difference in stored energy fuel, it doesn’t mean that you will become regularly depleted, expend all energy during training or need that amount of energy fuel all the time. With many things in training, and even nutrition, it all depends. However, how quickly glycogen stores might be depleted depends on the duration and intensity of the exercise and training session(s). Therefore, when it comes to fuel use during training, it’s all about that intensity and duration.
Fuel Breakdown 101
The relative utilization of fat and carbohydrates during exercise can vary enormously and depends strongly on exercise intensity. In the current training and nutrition culture, there is still a large majority of those who are die-hard carb fans or die-hard fat fans. They demonize each other because of what they “believe” is the better fuel for training. It isn't “what’s the best fuel” but rather “when,” “how,” and “why” that matters most. It’s important to keep in mind that the fuel use and exercise intensity phenomenon was originally based on V02 max (7). However, because most of us care about lifting, the same information and physiology apply to your training as well.
During exercise, carbs and fats are the main source of fuel, not protein. Although protein can be used in the absence of low glycogen and long, extended bouts of training from endurance exercise via glycogenolysis, carbs and fats are the main players. There is a mixture of fat and carbohydrate utilization during training, as the relative contribution of fat and carbohydrate utilization to total substrate metabolism is dependent on exercise intensity, exercise duration and dietary and training status (6, 8, 19).
For the sake of simplicity, carbohydrates are stored in the body as muscle glycogen and liver glycogen and circulated as plasma glucose. Interestingly, the liver has the highest concentration of glycogen (stored carbs), but by virtue of its size, skeletal muscle contains the largest store of glycogen (8). Glycogen provides an additional source of glucose besides that produced via gluconeogenesis (i.e. creation of new glucose from non-carb sources like protein). To help you better understand, glycogen contains several glucoses because it acts like a battery backup for the body, providing a quick source of glucose when needed and supplying a place to store excess glucose when glucose concentrations in the blood increase. In addition, glycogen is broken down from the "ends" of the molecule as more branches translate to more ends. More glucose can then be released at once for the liver and especially skeletal muscle during training sessions.
To keep things rolling, the breakdown of glycogen involves the release of glucose-1-phosphate (G1P), the rearranging of the remaining glycogen (as necessary) to permit continued breakdown and the conversion of G1P to glucose-6-phosphate (G6P) for further metabolism. As you may recall from your bioenergetics or exercise physiology class, G6P can be broken down during glycolysis, converted to glucose by gluconeogenesis and oxidized by another pathway (pentose phosphate pathway) that parallels glycolysis.
Fat is stored as adipose tissue (which is now considered a hormone) and as intramuscular triglyceride (IMTG). In addition, some fat is present in the circulation as plasma free fatty acids (FFA) and as triglycerides incorporated in lipoproteins (biochemical molecules classified by their density that contain both fat and protein and allow fat to move in and out of the cell). Common lipoproteins include VLDL, LDL and HDL.
As you may recall from part 1, fat oxidation requires oxygen. Because fats are long carbon chains, they are transported to the muscle mitochondria where the carbon atoms are used to produce acetyl-CoA. In a four-step process known as beta-oxidation, fatty acids are broken down into acetyl-CoA to form CO2 and H2O.When using fat, triglycerides are first broken down into free fatty acids and glycerol (a process called lipolysis). The breakdown of fatty acid compounds goes directly into the mitochondria to produce ATP, as the oxidation of free fatty acids generate considerably more ATP molecules than the oxidation of glucose or glycogen. On an interesting side note, in order to transport fatty acids across the mitochondria, this breakdown requires what’s called a carnitine-dependent transport system or shuttle (6, 13). In case you didn’t know, this is the location and main rationale for supplementing with L-carnitine and why carnitine is a popular ingredient in fat burner products (16, 23, 30).
Fuel Use and Training Intensity
Now that you have a basic idea of fuel breakdown, we'll get started with the meat and potatoes. Keep in mind that in these next several sections, fuel use and training intensity were originally built on V02 max. However, make no mistake. It 100 percent applies to lifting and resistance training. As exercise intensity increases from rest to near maximal levels, there is a gradual transition to use more glucose and glycogen as the predominant sources of ATP (19). From a metabolic perspective in the mitochondria, more ATP can be produced aerobically from the breakdown of carbohydrate as opposed to fat. However, the main determining factors are exercise duration and training intensity. Other factors include age, training, diet, gender and hormonal factors (15).
In the post-absorptive state, the majority of energy utilization comes from fat. During low intensity exercise (25–50 percent V02 max, range of motion, activation and movement preparation or RAMP for lifting), the majority of energy production stems from lipid oxidation, as plasma free fatty acid (FFA) oxidation provides most of the energy needed. At lower exercise intensities, most of the fatty acids used during training come from the blood (14). As exercise increases to moderate intensity (around 60 percent of VO2 max), the majority of fatty acids oxidized appear to come from intramuscular triglyceride (14). From a lab perspective, the technical term to measure energy expenditure and fuel use is respiratory exchange ratio (RER). The RER is a numeric index of carbohydrate and fat utilization based on a ratio of carbon dioxide produced to oxygen consumed. When using a metabolic cart, 0.7 is deemed 100 percent fat usage, 0.85 is a mix of fat and carbs and 0.85 or greater up to 1.0+ is pure carb metabolism (19, 22). However, these indexes aren't always straight forward, as those with certain metabolic abnormalities (i.e. diabetes) and trained athletes elicit different responses and do vary considerably.
During training, there is a dramatic increase in energy requirements because of the metabolic demand of working muscles. Therefore, at greater intensities (i.e. > 60 percent VO2 max or completion of movement preparation and the first several sets of lifting, volume work and heavier weights), the absolute contribution of fat oxidation reaches maximum rates, thus transitioning energy production needed for exercise to carbohydrate utilization. As the intensity of exercise increases, there is a significant reduction in lipid oxidation and a significant increase in carbohydrate metabolism. This phenomenon of the influence of exercise intensity and substrate utilization is termed the “crossover point/concept.” It was first developed by Brooks and Mercier (7) and simply describes the crossover effect from fat metabolism to more prominent carbohydrate metabolism (figure 1).
Figure 1: Crossover effect
As previously mentioned, even though this concept was originated from VO2 max, it 100 percent applies to lifting. The crossover point is the intensity (expressed as a percentage of VO2 max but also applies to heavy strength training) where fats and carbohydrates intersect and the energy from fats is reduced and the energy from carbohydrates is increased. With increased exercise intensities, there are many processes that contribute to the increased usage of carbohydrates.
These include:
- Contraction induced glycogenolysis (breakdown of glycogen): As the intensity increases, glycogen breakdown occurs via specific pathways (i.e. calcium/calmodulin), and epinephrine (adrenaline) catalyzes the formation of cyclic adenosine monophosphate (or cAMP), a second messenger important in many biological processes and derived from ATP that stimulates certain enzymes and glycogen breakdown.
- Fast twitch motor units: As exercise intensity increases, many more fast twitch muscle fibers are recruited that are much more suited (because of their enzymes) to utilize carbohydrates for the needed ATP production. As intensity increases, so does the glycolytic rate/flux along with energy demand and force output (i.e. Olympic lifting, speed deadlifts, max effort bench). Fast twitch muscle fibers are recruited in order to maintain/increase overall power output levels for that specific exercise in addition to an increase in overall sympathetic nervous system activity.
- Epinephrine: Higher intensity exercise stimulates epinephrine production (adrenaline), which also enhances carbohydrate metabolism (19). As intensity increases, there is an increase in epinephrine, as epinephrine intensifies the contraction-induced rate of muscle glycogenolysis (breakdown of glycogen).
- Lactate:As intensity increases, the glycolytic rate also increases along with the glycolytic enzymes, thus increasing the formation of lactate. Lactate inhibits free-fatty acid mobilization and thus decreases uptake into the muscle. The increased lactate production, due to an increased proton accumulation, inhibits hormone sensitive lipase (or HSA enzyme). Free fatty acids aren't released into circulation and become trapped in the adipose tissue. These overall activities inhibit lipolysis and fat breakdown.
- Blood flow:The reduction in fat utilization during high intensity training is also related to blood flow. Although blood flow increases to the overall working muscle during training and low intensity exercise, blood flow to adipose tissue is decreased during high intensity efforts. Thus, there is a reduced fatty acid supply to the muscle. In other words, at high exercise intensity, blood flow is directed away from adipose tissue so that fatty acids released from adipose tissue become “trapped” in the adipose capillary beds and, consequentially, aren't carried to the muscle to be used.
Although these events are more complex than explained, you get the idea. These combinations of events all lead to a profound increase in carbohydrate metabolism and reduced lipid metabolism during high intensity exercise.
Before anybody goes nuts, there are some differences between carbs and fats with respect to energy provisions.
The primary advantages of stored carbohydrates in animals and humans are:
- Energy isn't released from fat as fast as from glycogen.
- Glycolysis provides a mechanism of anaerobic metabolism (see part 1). This is important for muscle cells that can't get oxygen as fast as needed.
- Glycogen delivers a means of preserving glucose levels that can't be provided by fat.
Don’t misunderstand and take this out of context. I’m not saying that fat isn't used or can't be used for energy because it can. The highest outputs of the body are from anaerobic metabolism, which can only run off either the phosphagen system or glycolysis. You can certainly train to get better at utilizing fat because it does provide some advantages, but you will never see the highest outputs from an individual fueled only by fat. Bioenergetically, it just doesn’t occur because the maximal rate of ATP generation is slower than that of glycogen oxidation and more than tenfold slower than that with creatine phosphate.
The critical part that nearly everybody misses is the rate at which the body can produce energy during training. It's just impossible to utilize fat to power fast, explosive movement; heavy, low rep work; and moderate to high rep work. As I alluded to in part one, a main disadvantage of anaerobic work is the production of other metabolic byproducts (i.e. H+ or protons) that prevent the sustained output over the course of training. However, from a training application, it’s best to train both (and preferably all energy systems) to their fullest extent.
Interestingly, a phenomenon that has gained enormous attention in recent years is the concept of metabolic flexibility (3, 4, 9, 11, 17, 18, 21, 31, 34). Although a whole other topic, it’s the ability to switch from one fuel source to the next in a continuous fashion, whereby having the ability to train to switch from one fuel to the next because insulin is the substrate regulator, or main fuel selector switch, that switches your body from burning mostly fat to mostly carbohydrate when needed (preferably during training periods). So does this mean that you need to eat a whole meat lovers' pizza before you train? No, but you do need to be cognizant of your training, nutritional habits and individual responses to training as well as how they vary depending on training and intensity type, duration, frequency and volume. Interestingly, our bodies' default metabolic state is one of fat oxidation, and insulin is simply the switch that turns it to carbohydrate metabolism when needed. However, many individuals and some athletes never switch fuel usage during training.
Fats for Energy and Recovery
Because we just discussed the effect of training and intensity on fuel usage, your next question is “what about fat?” If you use more carbs during training because of the intensity, what about fat? Are you burning fat during training or during recovery? And when does it occur and how? After all, when it comes to fat loss and getting leaning, energy expenditure, a caloric deficit (for the most part) and a customized nutrition plan are the name of the game.
Isn’t it ironic that at low intensities (and long durations like long, slow cardio), you achieve a greater relative percentage of calories used from fat storage versus higher intensities? But higher exercise intensities attained from interval training, metabolic resistance training, training volumes, total body workouts, sprints, Prowler sprints, sled drags, supersets, tri-sets, giant sets and complexes not only expend more energy but also increase the body’s potential to use fats as an energy substrate to a greater extent than steady-state aerobic exercise and traditional resistance training due to increased up-regulation of enzymes responsible for beta-oxidation (process of fat breakdown). Further, total caloric expenditure and lipolysis (fat breakdown) are greatly enhanced by resistance training (26, 28, 32, 33) and higher intensity types of training, resulting in significant effects on fat loss (32).
Here’s the deal—because of the complex nature of the human body, it continually adjusts its use of fat for fuel. However, as mentioned in the previous sections, substrate utilization is highly governed by many factors including hormonal regulation, enzyme activity and genetic influences, and these factors can change by the moment (29). Many get caught up in how much fat they burn in a given training session. The truth is in order to obtain any significant effect and keep perspective on body composition, fat burning must be considered over the course of days, weeks or months, not on an hourly basis (12) and not during a single training session. Inevitably, the more carbs you expend during training, the more fat you burn in the post-exercise period and vice versa. This elevated metabolic rate is the concept and application of excess post-exercise oxygen consumption (EPOC). This occurrence can burn additional fat and calories up to 72 hours post-training, with intensity and duration (especially intensity) being the presiding factors [figure 2 (5, 20)] as well as replenishment of creatine phosphate, the metabolism of lactate, temperature recovery, heart rate recovery, ventilation (breathing) and hormonal factors. Further, EPOC has been notably observed after resistance training (25), resulting in a larger energy requirement after exercise to restore the contracting muscle cells to pre-exercise levels. EPOC has also been shown to be elevated for an extended period of time following eccentric training as well (10) due to the energy cost associated with protein synthesis and the repair and regeneration process. In addition, during the period of EPOC, fat oxidation rates have also been shown to increase (1, 2, 25).
Figure 2: Effects of EPOC and exercise intensity
Highly trained individuals and athletes have a much better ability to utilize fats and carbs during training, and even during recovery periods, and have a greater affinity to switch fuel usage for training and non-training days (i.e. metabolic flexibility). Adaptations that enhance fat usage in trained muscles involve improving fatty acid availability to the muscle and mitochondria and improving the ability to oxidize fatty acids. These adaptations lie largely within endurance training. However, in recent years, they have transitioned over to lifting and other competitive sports (i.e. fat adapt). From a nutritional perspective, this has to do with the concept on fat adaptation.
“Fat adaptation” is a protocol in which mainly endurance athletes consume a high fat, low carb diet for up to 14 days while undertaking their normal training [both high volume and high intensity (40)]. It can also be used as a stand-alone dietary strategy or can be followed immediately by a period of CHO restoration (consuming a high carb diet and tapering for 1–3 days). Further, there's a lot of controversy regarding the specific approaches to take and which fuel source people believe to be the “best.” Many feel that fat should be the preferred fuel source over carbs for training because they believe it enhances performance to a greater extent while others, and some scientific evidence, say otherwise. Of course, this is highly variable for each person, population (i.e. diabetes, fat loss clients, athletes), sport, individual responses to training and nutrition, goals and genetics. There is no one size fits all approach.
Due to these physiological occurrences in training discussed in this article, you can now begin to understand the idea and real life applications of how energy intake can influence substrate oxidation as well as the importance of nutrient timing and its affect on muscle strength, hypertrophy and protein synthesis, all of which govern and affect the large implications of sports performance.
There won't be a third article in this series unless requested by readers, trainers or coaches. If you have a desire to learn about another aspect of energy systems and applications or have ideas, please feel free to address them.
References
- Achten J, Jeukendrup AE (2003) Maximal fat oxidation during exercise in trained men. Int J Sports Med 24:603–8.
- Achten J, Jeukendrup AE (2004) Optimizing fat oxidation through exercise and diet. Nutrition 20:716–27.
- Bergouignan A, Antoun E, Momken I, Schoeller DA, Gauquelin-Koch G, Simon C, Blanc S (2013) Effect of contrasted levels of habitual physical activity on metabolic flexibility. J Appl Physiol (1985) 114:371–79.
- Bickel PE (2004) Metabolic fuel selection: the importance of being flexible. J Clin Invest 114:1547–49.
- Borsheim E, Bahr R (2003) Effect of exercise intensity, duration and mode on post-exercise oxygen consumption. Sports Med 33:1037–60.
- Brooks G, Fahey, Baldwin (2005) Exercise Physiology: Human Bioenergetics and Its Application.
- Brooks GA, Mercier J (1994). Balance of carbohydrate and lipid utilization during exercise: the "crossover" concept. J Appl Physiol (1985) 76:2253–261.
- Farrell J, Caiozzo (2012) ACSM's Advanced Exercise Physiology. 2nd ed. Lippincott Williams & Wilkins.
- Galgani JE, Moro C, Ravussin E (2008) Metabolic flexibility and insulin resistance. Am J Physiol Endocrinol Metab 295:E1009–17.
- Hackney KJ, Engels HJ, Gretebeck RJ (2008) Resting energy expenditure and delayed-onset muscle soreness after full-body resistance training with an eccentric concentration. J Strength Cond Res 22:1602–9.
- Hall KD (2010) Mechanisms of metabolic fuel selection: modeling human metabolism and body-weight change. IEEE Engineering in Medicine and Biology Magazine: The Quarterly Magazine of the Engineering in Medicine & Biology Society 29:36–41.
- Hansen K, Shriver T, Schoeller D (2005) The effects of exercise on the storage and oxidation of dietary fat. Sports Med 35:363–73.
- Holloway GP, Luiken JJ, Glatz JF, Spriet LL, Bonen A (2008) Contribution of FAT/CD36 to the regulation of skeletal muscle fatty acid oxidation: An overview. Acta Physiol (Oxf) 194:293–309.
- Horowitz JF, Klein S (2000) Lipid metabolism during endurance exercise. Am J Clin Nutr 72:558S–563S.
- Isacco L, Duche P, Boisseau N (2012) Influence of hormonal status on substrate utilization at rest and during exercise in the female population. Sports Med 42:327–42.
- Jeukendrup AE, Randell R (2011) Fat burners: nutrition supplements that increase fat metabolism. Obesity Reviews: An Official Journal of the International Association for the Study of Obesity 12:841–51.
- Kelley DE (2005) Skeletal muscle fat oxidation: timing and flexibility are everything. J Clin Invest 115:1699–1702.
- Kelley DE, Mandarino LJ (2000) Fuel selection in human skeletal muscle in insulin resistance: a reexamination. Diabetes 49:677–83.
- Kraemer WJ, Fleck, SJ, Deschenes MR (2012) Exercise Physiology: Integrating Theory and Application. Wolters Kluwer/Lippincott Williams & Wilkins: Philadelphia.
- LaForgia J, Withers RT, Gore CJ (2006) Effects of exercise intensity and duration on the excess post-exercise oxygen consumption. J Sports Sci 24:1247–264.
- Litvin M, Clark AL, Fisher SJ (2013) Recurrent hypoglycemia: boosting the brain's metabolic flexibility. J Clin Invest 123:1922–924.
- McArdle K, Katch (2009) Exercise Physiology: Energy, Nutrition, and Human Performance.
- Muller DM, Seim H, Kiess W, Loster H, Richter T (2002) Effects of oral L-carnitine supplementation on in vivo long-chain fatty acid oxidation in healthy adults. Metabolism 51:1389–391.
- Noakes T, Volek JS, Phinney SD (2014) Low-carbohydrate diets for athletes: What evidence? Br J Sports Med 48:1077–78.
- Ormsbee MJ, Choi MD, Medlin JK, Geyer GH, Trantham LH, Dubis GS, Hickner RC (2009) Regulation of fat metabolism during resistance exercise in sedentary lean and obese men. J Appl Physiol (1985) 106:1529–537.
- Ormsbee MJ, Thyfault JP, Johnson EA, Kraus RM, Choi MD, Hickner RC (2007) Fat metabolism and acute resistance exercise in trained men. J Appl Physiol (1985)102:1767–72.
- Paoli A, Rubini A, Volek JS, Grimaldi KA (2013) Beyond weight loss: a review of the therapeutic uses of very-low-carbohydrate (ketogenic) diets. Eur J Clin Nutr 67:789–96.
- Petitt DS, Cureton KJ (2003) Effects of prior exercise on postprandial lipemia: a quantitative review. Metabolism 52:418–24.
- Sonko BJ, Fennessey PV, Donnelly JE, Bessesen D, Sharp TA, Jacobsen DJ, Jones RH, Hill JO (2005) Ingested fat oxidation contributes 8% of 24-h total energy expenditure in moderately obese subjects. J Nutr 135:2159–165.
- Stephens FB, Constantin-Teodosiu D, Greenhaff PL (2007) New insights concerning the role of carnitine in the regulation of fuel metabolism in skeletal muscle. J Physiol 581:431–44.
- Storlien L, Oakes ND, Kelley DE (2004) Metabolic flexibility. Proc Nutr Soc 63:363–68.
- Tremblay A, Simoneau JA, Bouchard C (1994) Impact of exercise intensity on body fatness and skeletal muscle metabolism. Metabolism 43:814–18.
- Treuth MS, Hunter GR, Weinsier RL, Kell SH (1995) Energy expenditure and substrate utilization in older women after strength training: 24-h calorimeter results. J Appl Physiol (1985) 78:2140–146.
- van de Weijer T, Sparks LM, Phielix E, Meex RC, van Herpen NA, Hesselink MK, Schrauwen P, Schrauwen-Hinderling VB (2013) Relationships between mitochondrial function and metabolic flexibility in type 2 diabetes mellitus. PLoS One 8:e51648.
- Volek J, Sharman M, Gomez A, Judelson D, Rubin M, Watson G, Sokmen B, Silvestre R, French D, Kraemer W (2004) Comparison of energy-restricted very low-carbohydrate and low-fat diets on weight loss and body composition in overweight men and women. Nutr Metab (Lond) 1:13.
- Westman EC, Feinman RD, Mavropoulos JC, Vernon MC, Volek JS, Wortman JA, Yancy WS, Phinney SD (2007) Low-carbohydrate nutrition and metabolism. Am J Clin Nutr 86:276–84.
- Wycherley TP, Brinkworth GD, Clifton PM, Noakes M (2012) Comparison of the effects of 52 weeks weight loss with either a high-protein or high-carbohydrate diet on body composition and cardio metabolic risk factors in overweight and obese males. Nutrition & Diabetes 2:e40.
- Wycherley TP, Buckley JD, Noakes M, Clifton PM, Brinkworth GD (2014) Long-Term Effects of a Very Low-Carbohydrate Weight Loss Diet on Exercise Capacity and Tolerance in Overweight and Obese Adults. J Am Coll Nutr 1–7.
- Yancy WS, Jr., Olsen MK, Guyton JR, Bakst RP, Westman EC (2004) A low-carbohydrate, ketogenic diet versus a low-fat diet to treat obesity and hyperlipidemia: a randomized, controlled trial. Ann Intern Med 140:769–77.
- Yeo WK, Carey AL, Burke L, Spriet LL, Hawley JA (2011) Fat adaptation in well-trained athletes: effects on cell metabolism. Appl Physiol Nutr Metab 36:12–22.
- Zajac A, Poprzecki S, Maszczyk A, Czuba M, Michalczyk M, Zydek G (2014) The effects of a ketogenic diet on exercise metabolism and physical performance in off-road cyclists. Nutrients 6:2493–2508.
For example: If I performed 6 sets of 3 reps starting at 80% and working to 96% of my 1RM with 5 minutes rest in between sets (total time of 36 minutes) is that considered high intensity for the purposes of this article even though I'm not breathing hard and my heart rate doesn't go very high?
Example 2: Doing 5 sets across with 70% of 1 rep max for sets of 6 with 1 minute rest between sets (total time 12 minutes). Is this more or less intensity than the first example I gave with regard to the articles meaning of intensity? With this workout my heart rate will be over 85% max heart rate most of the training time, but the load is a lot less, the % of my 1rm is a lot lower (what I think of as intensity).
Thanks.
In your second example, 5 x6 @70% with 1 min would be technically be less intensity (lower 1RM) but many times the overall volume of work done is what separates things. Many can do sets of 1-3 and not get gassed but when you start doing reps that's what makes the difference in intensity (degree of effort) which is why your HR is higher for the 2nd example than the first. Make sense?
Which of these will cause a higher EPOC?
6 sets of 3 reps building up to a max triple with a PER of 10 on the last set (7 and 8 on previous)
5 sets of 5 @ 75% with a PER of 10 on the last set
In the first example the intensity (%1RM) is higher but the volume lower. In the second the volume is higher but the intensity (%1RM) is lower. In both cases the last set was everything the lifter had. the previous sets weren't as difficult. so which would you expect to cause more EPOC? or are they both roughly equal?
You mentioned that after exercise (EPOC) there is a shift towards fat oxidation. I read one study that said that fat oxidation is not completely surpressed post work out even when carbs come in. How can this be? Is this because of EPOC, catecholamines or other factors that help lipolysis even when insulin is arround?
thx