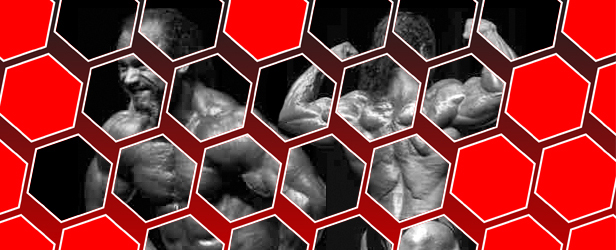
Have you committed an unnatural act today?
Morgan Freeman’s character in the movie Million Dollar Baby (2004) says “boxing is an unnatural act.” Believe it not, I frequently say the same about lifting weights, tongue in cheek of course, when asked why more people don’t exercise regularly. (Actually, the question is usually more along the lines of “why don’t people get off their lazy asses?”)
Exercise adherence issues aside, anything—a brutal training session, a “natural disaster,” a Frankensteinian creation of genetic engineering—that actually happens could be considered de facto “natural.” As far as weight training goes, unless you’re routinely arranging furniture per the whim of a highly indecisive interior decorator, the act of repeatedly picking up and putting down the very same object in the very same place over and over isn’t exactly an “activity of daily living,” at least for most people.
Is vitamin C an unnatural supplement?
Pondering many exercise-related activities from this semi-evolutionary, teleological viewpoint might lead one to think they are a bit “unnatural.” Drinking iced, calorically-laden recovery supplements during a workout is a bit counterintuitive but nonetheless a potentially effective bodybuilding strategy. (I cover this in my first set of articles.) In sporting competition, the delineation of what constitutes “natural” generally revolves more around what enhances performance versus what is man-made or not “natural” in that sense.
My focus here, though, is specifically on antioxidant supplementation and vitamin C in particular. The daily consumption of vitamin C in amounts equivalent to that of several dozen oranges (1g/day or more) is permitted in many naturally sporting organizations and fairly common despite dubious health(1–5) and performance benefits(6–8). Is that level of vitamin supplementation more “natural” than medically supervised restoration of blood testosterone to normal levels (for the purposes of health and well-being), a practice typically prohibited by overseeing sporting bodies that test for performance enhancing drugs (9–11)?
Semantics aside, let’s investigate whether megadosing vitamin C (otherwise known as ascorbic acid) is indeed an “unnatural act” from a biological perspective or simply a crafty application of sports nutritional technology.
A bit about vitamin C and free radicals
Ascorbic acid is both an antioxidant (quencher of free radicals) as well as a vitamin, at least for humans. A free radical is simply a reactive atom or molecule with one or more unpaired electrons that is highly likely to oxidize other molecules and thus create what’s called oxidative stress (12). As an antioxidant, vitamin C has the ability to counter the damaging effects of free radicals (13) implicated in a host of diseases (14) ranging from gastrointestinal disorders (15, 16) to cancer (17, 18) and even neurodegenerative conditions like Alzheimer’s (19). Free radicals may originate from unwanted sources such as radiation or toxins as well as normal biochemical processes within the body (17, 19). Of particular interest to you and me is that exercise actually generates free radicals and oxidative stress, in particular superoxide radicals (20, 21). However, as you’ll see below, this is the kind of stress we can maybe live with.
For humans, ascorbic acid is also a vitamin, meaning that it can't be produced endogenously (22)—we have to obtain it in our diets. The few mammals that lack this ability generally compensate with higher levels of an enzyme with similar antioxidant roles (superoxide dismutase). However, vitamin C is still needed because it does such a good job at protecting against oxidative damage to lipids and proteins and is particularly important for protecting the collagenous protein (23) found in structures like tendons, cartilage, and the skin (24).
How much “C” do I need to get an A?
Despite the role of oxidative stress in disease (5), research generally doesn’t support vitamin C supplementation for living a longer life (1, 2), although it may have a modest effect against some cancers (3). Some of this paradox is probably a matter of dose and the fact that vitamin C can act as a pro-oxidant (increasing oxidative stress), particularly if one consumes more than about 500mg/day(25–27). You see, the highest RDA for vitamin C (for lactating mothers) is a mere 120mg/day. (RDA for men 19 years old and above is just 90 mg (28).) A miniscule daily intake of only 10 mg of vitamin C is enough to prevent scurvy, which manifests in the skin due to collagen breakdown (29).
The rationale that we need or could make use of more vitamin C (in megadoses far beyond the RDA) largely ignores our bodies’ own ability to adjust free radical defenses (see below) and instead presumes that other animals’ ability to upregulate vitamin C biosynthesis should set our standard for vitamin C supplementation. Healthy, unstressed rats produce about 2 mg of vitamin C per 100 mg of body weight daily (or about 2 g/day for a 220-pound human sized “rat”) but can increase vitamin C production fivefold under toxic stress (30). However, when this baseline, unstressed vitamin C production is appropriately scaled from rat to human, based on the metabolic rate (31, 32), the equivalent is only about 300 mg per day, comfortably below the 500 mg/day level that some have suggested may lead to pro-oxidant effects (26).
Naturally, it’s possible that some individuals might need more antioxidant protection due to the oxidant stresses they encounter (5). If you’re thinking ahead, you might even suspect that there is even a time when providing antioxidant support could be especially beneficial, such as during a workout when one is producing more free radicals.
Stress is good?
If you’ve read my articles on nutrient timing during exercise in the form of a peri-workout recovery supplement, I may be about to throw you for a loop. Just as rats and most other animals can increase vitamin C in response to a free radical or toxic threat (23, 30), humans do so with the plethora of other components of our antioxidant defense system. Indeed, there is a common response element (an “on” switch) associated with a multitude of the genes involved in generating a counter-response to oxidative stress (33). This means that a single oxidative threat can quickly trigger a multifaceted and coordinated effort to quench free radicals (34). Mother ature didn’t leave us out in the cold when we lost the ability to synthesize vitamin C (35).
In fact, it seems as if oxidative stress is actually an essential part of engaging and coordinating intracellular free radical quenching. This kind of stress reactivity, termed hormesis (36, 37), is the body’s way of fine tuning its antioxidant army. The critical issue lies with what the appropriate amount of oxidative signaling is to improve antioxidant defenses without falling prey to the ill effects of oxidative damage.
Here’s the kicker
When it comes to exercise, oxidative stress is also crucial for eliciting training adaptation. In other words, by limiting oxidative stress during exercise, high dose antioxidant supplementation (with vitamin C or some other antioxidants) may put the kibosh on your training efforts. By analogy, excessively quenching free radicals during exercise may be like adding 200 pounds of upward band assistance to a bar only loaded to 200 pounds. It defeats the purpose. If you minimize training stress, you minimize adaptation.
Vitamin C, along with vitamin E, is the most studied culprit in this regard (20, 38-40). A daily dose of 1000 mg of vitamin C has been shown to prevent the normal increase in muscle mitochondria from endurance exercise (41). Add 400 IU of vitamin E to that dose of vitamin C and you may completely block the induction of the body’s antioxidant defense systems and even prevent the training-induced improvements in insulin sensitivity (42). However, more conservative daily dosing of vitamin C (500 mg) with E (400 IU) may not be enough to impact improvements in VO2 max (aerobic power) or mitochondrial density (43).
Where’s the kicker?
There is some disagreement in the literature regarding the effects of vitamin C and vitamin E on exercise training adaptation. In addition to dose, species differences (rat versus human), the possible pro-oxidant effects of high dosing (see above), subject training status, and even ineffectiveness of some supplement regimes to actually improve antioxidant status (redox homeostasis) are likely candidates for the mixed results in the research literature (40, 44). However, at very least, when it comes to exercise training adaptation, high dose vitamin C and vitamin E supplementation present long-term negatives that don’t seem to be balanced by tangible benefits (45).
Not all antioxidants are created equal
Because free radicals are both a signal for stress and adaptation, one might correctly suspect that they are also a potential source of muscle fatigue (46, 47). This may be partly because free radicals initiate oxidative damage during exercise itself (48, 49). Quenching free radicals with an antioxidant like coenzyme-Q10 may indeed be ergogenic during a single bout (50), but by limiting the training signal, doing so also impairs adaptation to repeated bouts (51). Similarly, n-acetylcysteine has been found to improve performance (52) but also blunt the adaptive processes after exercise (53). In one study, 14 days of vitamin C dosing (1g/day) did not reduce post-exercise muscle soreness (after a single damaging bout of downhill running) but impaired the full recovery muscle strength, which manifested after the first week of supplementation (54).
Luckily for you and me, not all antioxidants are created equal. Some, in fact, are known to exert their effects by calling into action our cells’ own antioxidant defenses. Quercetin has this effect (55) and has been shown to improve aerobic exercise performance and stimulate mitochondrial biogenesis (1000mg/day) after only two weeks of supplementation (56).
Whey protein, if undenatured (57), is truly impressive in this regard, as it has powerful intrinsic antioxidant activity, making it ergogenic (58, 59) in both rodents (60, 61) and humans(62). More importantly though, undenatured whey consumption increases activity of the body’s own free radical scavenging enzymes in animals (58) and people (63–65).
Nature has provided us with a multitude of other substances like quercetin and undenatured whey that upregulate cells’ ability to quench free radicals. This property has also been demonstrated in plant extracts (e.g., from white willow bark (66) to several food components and spices (67) including cinnamon (68–70), garlic (71–73), curcumin (found in tumeric), carnosol (rosemary), sulforaphane (cruciferous vegetables like broccoli), and polyphenols such as resveratrol (Japanese knotweed), quercetin (various fruits and vegetables) and EGCG (green tea) (18, 55)).
Go natural with super supplements
It’s unknown just where the tipping point lies (5) between free radical stress that encourages adaptive defenses (40) and free radical quenching that prevents oxidative stress and its correlates of disease and aging (37). Perhaps ergogenic antioxidants could be strategically and only periodically used, thus avoiding interfering with training adaptation. (For example, one might use them to prevent overtraining during high volume or high intensity training phases.) A better option, from a mechanistic standpoint as well as from the limited existing data, is to employ antioxidant “super supplements” such as those listed above to encourage expression of the body’s own endogenous antioxidant activity, thus truly “supplementing” and reinforcing the natural cellular adaptation to oxidative stress.
When it comes to vitamin C and E (and the same may hold true for coenzyme-Q10, N-acetylcysteine, and other similar antioxidants), some researchers have gone so far as to say that these “antioxidant supplements are, at the least, useless" (45).
In other words, you could even say that megadosing of antioxidant vitamins like C and E is an “unnatural act.” Regardless of terminology, the bottom line is that regularly doing so may very well run counter to getting the results from your training that you so rightly deserve.
References
- Bjelakovic G, et al (2007) Mortality in randomized trials of antioxidant supplements for primary and secondary prevention: systematic review and meta-analysis. JAMA 297(8):842–57.
- Bjelakovic G, et al (2012) Antioxidant supplements for prevention of mortality in healthy participants and patients with various diseases. Cochrane Database Syst Rev 3:Cd007176.
- Patterson RE, et al (1997) Vitamin supplements and cancer risk: the epidemiologic evidence. Cancer Causes Control 8(5):786–802.
- Evans JR, Lawrenson JG (2012) Antioxidant vitamin and mineral supplements for slowing the progression of age-related macular degeneration. Cochrane Database Syst Rev 11:Cd000254.
- Willcox JK, et al (2004) Antioxidants and Prevention of Chronic Disease. Critical Reviews in Food Science and Nutrition 44(4):275–95.
- Connolly DA, et al (2006) The effects of vitamin C supplementation on symptoms of delayed onset muscle soreness. J Sports Med Phys Fitness 46(3):462–7.
- Thompson D, et al (2001) Muscle soreness and damage parameters after prolonged intermittent shuttle-running following acute vitamin C supplementation. Int J Sports Med 22(1):68–75.
- Bryer SC, Goldfarb AH (2006) Effect of high dose vitamin C supplementation on muscle soreness, damage, function, and oxidative stress to eccentric exercise. Int J Sport Nutr Exerc Metab 16(3):270–80.
- Page ST, et al (2005) Exogenous testosterone (T) alone or with finasteride increases physical performance, grip strength, and lean body mass in older men with low serum T. J Clin Endocrinol Metab 90(3):1502–10.
- Corona G, et al (2013) Risks and benefits of late onset hypogonadism treatment: an expert opinion. World J Mens Health 31(2):103–25.
- Bassil N, et al (2009) The benefits and risks of testosterone replacement therapy: a review. Ther Clin Risk Manag 5(3):427–48.
- Sies H (1997) Oxidative stress: oxidants and antioxidants. Exp Physiol 82(2):291–5.
- Fisher-Wellman K, Bloomer RJ (2009) Acute exercise and oxidative stress: a 30 year history. Dyn Med 8:1.
- Wahlqvist ML (2013) Antioxidant relevance to human health. Asia Pac J Clin Nutr 22(2):171–6.
- Aleksunes LM, Manautou JE (2007) Emerging role of Nrf2 in protecting against hepatic and gastrointestinal disease. Toxicol Pathol 35(4):459–73.
- Bataille AM, Manautou JE (2012) Nrf2: a potential target for new therapeutics in liver disease. Clin Pharmacol Ther 92(3):340–8.
- Zhang Y, Gordon GB (2004) A strategy for cancer prevention: stimulation of the Nrf2-ARE signaling pathway. Mol Cancer Ther 3(7):885–93.
- Zhao CR, et al (2010) Nrf2-ARE signaling pathway and natural products for cancer chemoprevention. Cancer Epidemiology 34(5):523–33.
- Joshi G, Johnson JA (2012) The Nrf2-ARE pathway: a valuable therapeutic target for the treatment of neurodegenerative diseases. Recent Pat CNS Drug Discov 7(3):218–29.
- Gomez-Cabrera MC, et al (2008) Moderate exercise is an antioxidant: upregulation of antioxidant genes by training. Free Radic Biol Med 44(2):126–31.
- Gomes EC, et al (2012) Oxidants, antioxidants, and the beneficial roles of exercise-induced production of reactive species. Oxid Med Cell Longev.
- Garrett RH, Grisham CM (1995) Biochemistry. Orlando: Harcourt Brace College Publishers, 1100.
- Chatterjee IB (1998) Vitamin C: Biosynthesis, evolutionary significance and biological function. Proceedings of the Indian National Science Academy B64(3):213–234.
- Guyton AC (1991) Textbook of medical physiology. 8th ed. Philadelphia: Saunders, xli–1014.
- Carr A, Frei B (1999) Does vitamin C act as a pro-oxidant under physiological conditions? The FASEB Journal 13(9):1007–24.
- Podmore ID, et al (1998) Vitamin C exhibits pro-oxidant properties. Nature 392:559.
- Kang SA, et al (1998) In vivo dual effects of vitamin C on paraquat-induced lung damage: dependence on released metals from the damaged tissue. Free Radic Res 28(1):93–107.
- United States Department of Agriculture (2011) DRI Tables for Vitamins and Minerals. Accessed September 13, 2011. Available from: http://fnic.nal.usda.gov/nal_display/index.php?info_center=4&tax_level=3&tax_subject=256&topic_id=1342&level3_id=5140.
- Padayatty SJ, et al (2003) Vitamin C as an antioxidant: evaluation of its role in disease prevention. J Am Coll Nutr 22(1):18–35.
- Conney AH, et al (1961) Metabolic interactions between L-ascorbic acid and drugs. Ann N Y Acad Sci 92:115–27.
- Sharma V, McNeill JH (2009) To scale or not to scale: the principles of dose extrapolation. Br J Pharmacol 157(6):907–21.
- Health USD, et al (2005) Guidance for Industry: Estimating the Maximum Safe Starting Dose in Initial Clinical Trials for Therapeutics in Adult Healthy Volunteers. Available from: http://www.fda.gov/downloads/Drugs/GuidanceComplianceRegulatoryInformation/Guidances/ucm078932.pdf.
- Wasserman WW, Fahl WE (1997) Functional antioxidant responsive elements., Proceedings of the National Academy of Sciences 94(10):5361–66.
- Ishii T, et al (2000) Transcription Factor Nrf2 Coordinately Regulates a Group of Oxidative Stress-inducible Genes in Macrophages. Journal of Biological Chemistry 275(21):16023–29.
- Nandi A, et al (1997) Evolutionary significance of vitamin C biosynthesis in terrestrial vertebrates. Free Radic Biol Med 22(6):1047–54.
- Ristow M, Zarse K (2010) How increased oxidative stress promotes longevity and metabolic health: The concept of mitochondrial hormesis (mitohormesis). Experimental Gerontology 45(6):410–18.
- Finkel T, Holbrook NJ (2000) Oxidants, oxidative stress and the biology of ageing. Nature 408(6809):239–47.
- Jackson MJ (2008) Free radicals generated by contracting muscle: by-products of metabolism or key regulators of muscle function? Free Radic Biol Med 44(2):132–41.
- Powers SK, et al (2010) Reactive oxygen species are signalling molecules for skeletal muscle adaptation. Exp Physiol 95(1):1–9.
- Nikolaidis MG, et al (2012) Does vitamin C and E supplementation impair the favorable adaptations of regular exercise? Oxid Med Cell Longev.
- Gomez-Cabrera MC, et al (2008) Oral administration of vitamin C decreases muscle mitochondrial biogenesis and hampers training-induced adaptations in endurance performance. The American Journal of Clinical Nutrition 87(1):142–49.
- Ristow M, et al (2009) Antioxidants prevent health-promoting effects of physical exercise in humans. Proc Natl Acad Sci USA 106(21):8665–70.
- Yfanti C, et al (2010) Antioxidant supplementation does not alter endurance training adaptation. Med Sci Sports Exerc 42(7):1388–95.
- Theodorou AA, et al (2011) No effect of antioxidant supplementation on muscle performance and blood redox status adaptations to eccentric training. Am J Clin Nutr 93(6):1373-83.
- Gomez-Cabrera MC, et al (2012) Antioxidant supplements in exercise: worse than useless? Am J Physiol Endocrinol Metab 302(4):E476–7.
- Reid MB (2008) Free radicals and muscle fatigue: Of ROS, canaries, and the IOC. Free Radical Biology and Medicine 44(2):169–79.
- Barclay JK, Hansel M (1991) Free radicals may contribute to oxidative skeletal muscle fatigue. Can J Physiol Pharmacol 69(2):279–84.
- Castrogiovanni P, Imbesi R (2012) Oxidative stress and skeletal muscle in exercise. Italian Journal of Anatomy and Embryology 117(2):107–17.
- Banerjee AK, et al (2003) Oxidant, antioxidant and physical exercise. Molecular and Cellular Biochemistry 253(1–2):307–12.
- Gökbel H, et al (2010) The effects of coenzyme Q10 supplementation on performance during repeated bouts of supramaximal exercise in sedentary men. Journal of Strength and Conditioning Research 24(1):97–102.
- Malm C, et al (1997) Effects of ubiquinone-10 supplementation and high intensity training on physical performance in humans. Acta Physiol Scand 161(3):379–84.
- Medved I, et al (2004) N-acetylcysteine enhances muscle cysteine and glutathione availability and attenuates fatigue during prolonged exercise in endurance-trained individuals. Journal of Applied Physiology 97(4):1477–85.
- Petersen AC, et al (2012) Infusion with the antioxidant N-acetylcysteine attenuates early adaptive responses to exercise in human skeletal muscle. Acta Physiol (Oxf) 204(3):382–92.
- Close GL, et al (2006) Ascorbic acid supplementation does not attenuate post-exercise muscle soreness following muscle-damaging exercise but may delay the recovery process. Br J Nutr 95(5):976–81.
- Hur W, Gray NS (2011) Small molecule modulators of antioxidant response pathway. Current Opinion in Chemical Biology 15(1):162–73.
- Nieman DC, et al (2010) Quercetin’s influence on exercise performance and muscle mitochondrial biogenesis. Med Sci Sports Exerc 42(2):338–45.
- Chen J, et al (2003) Antioxidant capacity of bovine milk as assayed by spectrophotometric and amperometric methods. International Dairy Journal 13(12):927–35.
- Liu J, et al (2011) Retard of Whey Hydrolysates Supplementation on Swimming Exercise-induced Fatigue in Mice. Asian Journal of Animal and Veterinary Advances 6:1214–23.
- Liu J, et al (2013) Effect of whey protein hydrolysates with different molecular weight on fatigue induced by swimming exercise in mice. J Sci Food Agric.
- Bounous G, Gold P (1991) The biological activity of undenatured dietary whey proteins: role of glutathione. Clin Invest Med 14(4):296–09.
- Bounous G, et al (1988) The immunoenhancing property of dietary whey protein concentrate. Clin Invest Med 11(4):271–8.
- Lands LC, et al (1985) Effect of supplementation with a cysteine donor on muscular performance. J Appl Physiol 87(4):1381–5.
- Balbis E, et al (2009) Whey proteins influence hepatic glutathione after CCl4 intoxication. Toxicol Ind Health 25(4–5):325–-8.
- Bounous G, et al (1993) Whey proteins as a food supplement in HIV-seropositive individuals. Clin Invest Med 16(3):204–9.
- Micke P, et al (2001) Oral supplementation with whey proteins increases plasma glutathione levels of HIV-infected patients. Eur J Clin Invest 31(2):171–8.
- Ishikado A, et al (2012) Willow bark extract increases antioxidant enzymes and reduces oxidative stress through activation of Nrf2 in vascular endothelial cells and Caenorhabditis elegans. Free Radic Biol Med.
- Balstad TR, et al (2011) Coffee, broccoli and spices are strong inducers of electrophile response element-dependent transcription in vitro and in vivo – studies in electrophile response element transgenic mice. Mol Nutr Food Res 55(2):185–97.
- Kim BH, et al (2010) Regulatory effect of cinnamaldehyde on monocyte/macrophage-mediated inflammatory responses. Mediators Inflamm 529–59.
- Wondrak GT, et al (2010) The cinnamon-derived dietary factor cinnamic aldehyde activates the Nrf2-dependent antioxidant response in human epithelial colon cells. Molecules 15(5):3338–55.
- Cabello CM, et al (2009) The cinnamon-derived Michael acceptor cinnamic aldehyde impairs melanoma cell proliferation, invasiveness, and tumor growth. Free Radic Biol Med 46(2):220-31.
- Fisher CD, et al (2007) Induction of drug-metabolizing enzymes by garlic and allyl sulfide compounds via activation of constitutive androstane receptor and nuclear factor E2-related factor 2. Drug Metab Dispos 35(6):995–1000.
- Zhang P, et al (2006) Different activation patterns of rat xenobiotic metabolism genes by two constituents of garlic. Carcinogenesis 27(10):2090–5.
- Yang CS, et al (2001) Mechanisms of inhibition of chemical toxicity and carcinogenesis by diallyl sulfide (DAS) and related compounds from garlic. J Nutr 131(3s):1041–5.